By Andrew Myers Illustrations by Andrew Colin Beck
In November 2014, a new sort of high-rise emerged on a grassy, 3-acre plot in East Baltimore, directly across from Johns Hopkins Bayview Medical Center.
This high-rise was not like any that had gone before. Its units, stacked upon one another, do not soar into the sky. Instead, they fit neatly inside a 3,786-square-foot building. Its “condos” require no homeowners’ fees. Its multicolored lights flicker around the clock, but only a handful of humans ever traipse the halls. In fact, the residents of this complex—spinning, swirling, associating, connecting—are not people at all, but individual bits of data.
The new structure is the gleaming home of the new Maryland Advanced Research Computing Center—“MAR-see,” for short— a $30 million joint effort of Johns Hopkins University and the University of Maryland, supported by the state of Maryland.
MARCC, in essence, is the queen of a 21st-century high-tech beehive in which light-speed fiber-optic connections whisk data to and from various campuses at 100 gigabits per second—10,000 times faster than your average home Internet connection.
At Johns Hopkins, where all researchers will access the center remotely, MARCC’s resources will be meted out among four schools: Engineering, Medicine, Public Health, and Arts and Sciences (home of the physics department). The Whiting School of Engineering will enjoy one of the largest shares of time—10 million hours per quarter, approximately 27 percent of the Johns Hopkins’ total allotment.
“MARCC really brings Johns Hopkins engineering to the forefront of advanced computing,” says Jaime Combariza, an associate research professor in chemistry and director of MARCC. “It’s really about ‘want’ versus ‘can’ in terms of what we are able to study.”
In its center, MARCC boasts more than 19,000 core processors, rivaling some of the largest and fastest computers in the country, if not the world. MARCC can execute half a trillion calculations per second—decoding the 3 billion “letters” of the human genome in, say, in a matter of hours, compared to the more than 10 years it took Francis Collins and Craig Venter in 2000.
MARCC has 17 petabytes of storage capacity. That’s 17 million gigabytes, enough to store 225 years of high-definition video footage. Its stacks require 1.5 megawatts of electricity, enough to power a small village, and must be cooled by air conditioners that are so loud that administrators and other visitors must wear protective headphones when they stride among the stacks.
Keeping it all humming along is Combariza’s job. Since coming online in the summer of 2015, MARCC has been overwhelmed by demand. Eight-five percent of its capacity is already claimed, but that doesn’t worry Combariza. There is enough land here for four additional centers of MARCC-like scale, he says. All he needs is funding to expand.
In the months—and years—ahead, MARCC will help crunch numbers of some very big data sets to quickly and efficiently answer some of the biggest questions the world has to offer.
When it comes to engineering, Rajat Mittal, a professor of mechanical engineering, is something of a Renaissance man. His interests focus largely on questions of fluid dynamics—how fluids behave— but the applications of his work range broadly, touching on everything from the aerodynamics of speech to blood flow in the heart.
“We are all living creatures. We live in fluids—air and water. Also, we are made up of fluids, inside body and out,” Mittal says of his expansive interests. “I study how animals live in fluids—how we survive, walk, swim, and fly.”
Instead of using a wind tunnel to model how a butterfly flies, for instance, he does it on the computer. An advanced tool like MARCC allows Mittal to explore and answer complex problems, moving from a mere microcosm of a larger question to whole-organ or whole-organism analysis.
In one example, he simulated how blood flows inside the heart chambers and in arteries to better understand heart disease and heart murmurs.
“We are beginning to understand what the doctor hears when putting a stethoscope to the chest. We are learning to correlate the sound to the disease,” Mittal explains. He has also studied blood flow and biochemistry to understand how blood clots form after heart attacks.
In another direction entirely, Mittal has explored the aerodynamics of insect flight to learn how they move, maneuver, and hover with such precision. The findings, while amounting to basic science today, might one day lead to robots that fly with the grace and agility of a moth or a butterfly.
Mittal describes MARCC as a “godsend.” He mentors several doctoral and graduate students, and he cannot divert crucial funds to purchase the necessary hardware for his lab. Instead, he has largely depended upon access to national-level facilities of the National Science Foundation, the Department of Energy, and NASA.
The competition for those facilities is fierce. One of his recent requests for 6 million hours of processor time was cut drastically. It sounds like a lot, but with potentially thousands of processors running simultaneously, the hours can get chewed up fast. In the end, Mittal had to settle for 2 million hours—a third of his need.
“The national facilities are not keeping pace. It’s choking our research,” he says.
With MARCC, Mittal can now answer bigger, more complex questions, and he can do it in much less time than ever before. He has not been shy about relying upon MARCC. “I’m a big user. During the beta testing, they gave us 800,000 hours. We used it up in four weeks,” he says.
Down the road, Mittal says he would like to develop a presurgical tool to analyze blood flow to improve heart surgeries—a sort of flight simulator for cardiologists. Someday, he hopes, such simulations could happen in real time. To do that, simulations need to get 1,000 to 10,000 times faster.
“A single simulation takes one exaflop— a billion-billion—calculations. It used to take a week to run a model,” Mittal says. “That’s exactly the kind of goal MARCC can really help us get closer to.”
Natalia Trayanova, the Murray B. Sachs Professor in Biomedical Engineering, creates sophisticated, down-to-the-cell models of the human heart, but not models of just any heart. She creates exact models for specific patients. The result is a personalized map of the heart—what she calls a Google heart—allowing doctors to zoom in on diseased tissue and then out to see the whole organ.
Her models are poised to help doctors better diagnose and treat heart defects and damage, making corrective interventions more precise, less expensive, and, in some cases, unnecessary.
“We do very exact models of the mechanisms of cardiac arrhythmias, basically representing disease caused by microdegradations inside the cells, but it’s very computationally intensive. That’s where MARCC comes in,” says Trayanova, who is a professor of biomedical engineering and a member of the Institute for Computational Medicine.
When a person suffers a heart attack, blood flow to the heart itself is cut off and heart tissue dies. If lucky, the patient survives, but a permanent reminder remains in the form of scar where the healthy tissue used to be.
The scar is not without consequence. It can disrupt the electrical signaling, causing life-threatening arrhythmias. Often, the course of action is to install a defibrillator, but this is not an ideal solution. Only 5 percent of heart attack survivors annually will go on to develop arrhythmias in their first year after the device implantation.
“That’s 19 out of 20 patients getting defibrillators who don’t need them. We can reduce that number.” Moreover, she notes: “Most who die of sudden cardiac death do not meet the current clinical criteria for defibrillators. We can also predict which patients who do not meet the clinical criteria for implantation actually need them. That will save lives.”
The models her team develops combine advanced medical imagery from MRI and CT scans taken from real patients to create true-to-life 3-D models of the damaged heart that comprise millions of elements.
The imagery alone can distinguish healthy muscle from scar tissue. But her advanced algorithms then take things a step further, allowing doctors to see what the imagery could never show.
Trayanova’s algorithms model everything from chemical reactions to the orientation of the muscle fibers to see how electrical signals propagate through the tissue. She then can predict where muscle tissue will block or conduct electric signals with every heartbeat. In other words, her models can see how scar tissue affects the normal function of the heart. Better yet, they can help suggest the best course of treatment.
She hopes, someday, to bring new tools to the bedside and make their use routine. If she succeeds, it will be largely thanks to MARCC, which, she says, lets her do what was once impractical or impossible.
In one recent real-world example, Trayanova received a call from cardiologists saying they had a patient on the way in. They wanted to conduct the invasive anti-arrhythmia treatment the next day.
“They sent us the scans—a real patient with real complications—and we calculated the treatment model in 19 hours using MARCC, in time to inform the surgery the next day,” Trayanova explains.
Between the Folds
Jeffrey Gray focuses on the proteins inside human cells and how they behave and interact with one another. “Proteins are the building blocks of life and the drivers of things that happen inside the cell,” says the professor of chemical and biomolecular engineering.
In particular, Gray studies antibodies, vaccines, and how they combine so that he can better understand why and how a vaccine works or, more specifically, why a certain vaccine is effective only for a certain population. In the end, his models could lead to rapid development of and more effective vaccines.
Instead of conducting experiments in a petri dish, Gray uses computers to capture and model all of the motions and energies of proteins to understand what they are doing and how they function.
The ultimate goal is to design new and better interactions between proteins. “We hope to change how biology works to beat disease or design nanostructures to accomplish important tasks in the body,” Gray says.
A protein is like a long piece of spaghetti. Inside the cell, it can flop and fold many different ways. The shape determines the properties of the protein, where it goes, and, most importantly, what it does inside the cell. MARCC’s computer enables Gray to run through those permutations much faster than he ever could with his lab’s own resources.
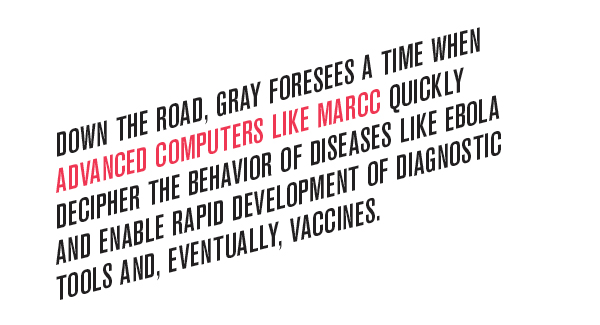
“MARCC is helping us do an end run on the structure of proteins. We can build a model with a few taps of the keys, see what’s most effective, and then adapt our models. MARCC lets us do this work three times as fast as before,” Gray says.
The number of processors dedicated to a problem determines the speed of his work. One machine might labor for three weeks to do a given simulation. Three hundred machines can do the same in a more reasonable 12 hours.
“MARCC can do it in 15 minutes,” Gray says. “We can do 100 cases in a matter of hours. Students can be changing modeling and design parameters and getting rapid results. It makes our feedback loop that much faster.”
Down the road, Gray foresees a time when advanced computers like MARCC quickly decipher the behavior of diseases like Ebola and enable rapid development of diagnostic tools and, eventually, vaccines.
“MARCC helps us to create better models and to apply them to some serious, real-world problems,” Gray says.
From the wonders of biology to the marvels of metal, MARCC is making its presence felt across the engineering spectrum, but you don’t need to tell that to Michael Falk, ’90 (A&S), MS ’91, a professor of materials science and engineering. He is a member of the Hopkins Extreme Materials Institute, otherwise known as HEMI.
MARCC’s base units are arranged in high-rise racks, which can be expanded over time. A half-rack is known at MARCC as a “condo.” HEMI, for instance, has plans to buy its own condo.
As a member of HEMI, Falk studies the properties of magnesium alloys for use in lightweight armor applications for the Army. He is trying to understand how magnesium reacts to stress and how to make it stronger.
“We use computers like MARCC to model the structure of various alloys and to analyze their mechanical properties—literally, what the strengths and weaknesses are when they’re twisted, bent, squeezed, or stretched,” Falk explains.
In his work, he deploys atomic-scale computational methods to increase his understanding of the processes that govern deformation, failure, and friction within and between materials. Magnesium, for example, has a very particular crystal structure that makes it brittle. But with the addition of various other metals—particularly aluminum—magnesium becomes stronger.
As with any models that approach simulation at the atomic level, the calculations necessary to capture accurately real-world performance become overwhelming for anything but the most advanced computer systems. In a short time, MARCC has had a profound effect on Falk’s work. MARCC lets him do in a few weeks what would have taken months before.
“We’re approaching the point where we can understand the plasticity of an alloy as a whole to create new and better materials,” Falk says.
The job of taking Falk’s atomic-level insights to broader scales is the province of researchers like Jaafar El-Awady, an assistant professor of mechanical engineering. His interests are in material deformation and failure, particularly for extreme environments in aerospace applications, such as turbine blades in jet engines.
When designing new metals for turbine engine blades, researchers traditionally have required years of trial and error to make improvements. That’s expensive.
Models allow engineers like El-Awady to test potential materials cheaply on a computer and to narrow down options. Only the most promising materials are accorded real-world tests.
“MARCC lets us do simulations that we only dreamed about before,” says El-Awady, who recently received a CAREER award from the National Science Foundation.
In the processing of materials, metals are made by growing layers of atoms atop one another. Ideally, the resulting crystal structure is perfect, but in reality it never is. Atoms do not always behave as expected and misalignments occur in the crystal structure, becoming weak points.
“Every material has significant number of defects,” El-Awady says. “They’re unavoidable.”
El-Awady explores how these defects move under high load and cause deformations that can compound, eventually leading to failure.
“The deformations create permanent markings—we call them slip bands—in the material that you can actually see,” El-Awady says. “Most fractures occur along these slip bands. We’re trying to predict from the microstructure level upward how the deformations evolve into a failure.”
Modeling large samples at the scale of an entire turbine blade would be impossible, even for a computer of MARCC’s capabilities.
“You would need to model each defect and microstructural feature in a sample. The number of calculations would be unimaginable,” El-Awady says. Instead, he uses a small-scale sample and simulates how it might perform as part of a larger sample. His models of larger materials are essentially models of models. That’s where MARCC is so invaluable.
“We’ve always known about slip bands, but we had been unable to model them,” El-Awady says. “MARCC is giving me and many others that capability. A system like this is really critical to the entire Hopkins scientific community.”