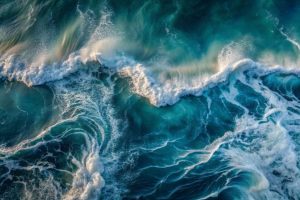
A team led by Johns Hopkins researchers has developed a mathematical model that sheds light on how small but crucial differences in the way water vapor travels through the atmosphere can lead to major shifts in ocean currents, potentially signaling “tipping points” in the Earth’s climate.
The team’s models, described in The Journal of Physical Oceanography, could enable a deeper understanding of climate dynamics and generate more accurate predictions—essential to developing strategies that can address and potentially even mitigate the impacts of climate change.
“Over the last thousand years, a pattern of ocean currents known as the ‘ocean conveyor belt” exchanges water between the surface and the deep ocean. Scientists are concerned about its breakdown, which could trigger significant climate shifts worldwide. Some hypothesized that similar changes caused the collapse of the Mayan civilization. But we don’t yet grasp the mechanisms behind these shifts,” said team member Ioannis Kevrekidis, Bloomberg Distinguished Professor in Applied Mathematics and Statistics and Chemical and Biomolecular Engineering at the Whiting School of Engineering.
“Our objective is to detect when we are on the brink of major shifts,” said study leader Anand Gnanadesikan, a professor in the Krieger School of Arts & Science’s Department of Earth and Planetary Sciences.
The researchers analyzed the geography of the Earth’s hydrological cycle, which transports water vapor in the atmosphere. Traditionally, it has been thought that the North Pacific receives more of this freshwater than the North Atlantic, making it the main location for transforming light surface waters into dense deep ones.
By combining stochastic considerations with advanced bifurcation theory—a method for analyzing how solutions to differential equations change with parameter variations—the team pinpointed critical points where ocean circulation patterns either collapse or restore. They found that there was a significant variety of potential configurations of the resulting conveyor belt circulations, as well as interesting dynamics driving the transitions between them.
Among their key findings was the sensitivity of Atlantic and Pacific Ocean currents to changes, depending on the characteristics of the water cycle, such as precipitation. By constructing a model that simulates the behavior of these currents under different hydrological cycle conditions, the researchers were able to identify various patterns and quantify their stability.
They found that increasing the water cycle’s intensity in their model caused an initial collapse of overturning in the Pacific, followed by a temporarily strengthened overturning in the Atlantic. However, as the water cycle strengthened further, a surprising transition occurred: the Atlantic circulation shut off, while the Pacific now started overturning to intermediate depths. When the hydrological cycle increased still further, both circulations collapsed.
“Although deep-sea sediment cores—samples of materials collected by drilling into the seabed—show evidence of such transitions happening in the past, this study is the first to predict and explain why they occur,” said Gnanadesikan.
The team also studied the impact of rising global temperatures and found that having Northern polar temperatures rise faster than Southern polar temperatures—as has occurred in historical times—in their models contributed to a significant disruption of the ocean’s vertical circulation, or overturning.
“Our work sheds light on how ocean currents behave and shows that different changes can lead to similar outcomes in ocean currents. The integration of mathematical modeling, advanced stochastic techniques, and climate science insights offers a powerful tool for understanding and predicting critical transitions in many complex systems,” Kevrekidis said.
JHU study co-authors included Thomas Haine, Renske Gelderloos, Marie-Aude Pradal, and undergraduate student Jingwen Liu—all of the Krieger School of Arts & Sciences’ Department of Earth and Planetary Sciences; and Jennifer Sleeman and Jay Brett of the Johns Hopkins University Applied Physics Laboratory. Gianluca Fabiani and Constantinos Siettos of the University of Naples were also collaborators.
“This work underscores the importance of collaboration between domain experts in climate science and applied mathematicians. It’s their deep insights into Earth’s oceans and atmosphere that provide the foundational principles driving our models”, Kevrekidis said.
The study was funded by DARPA.