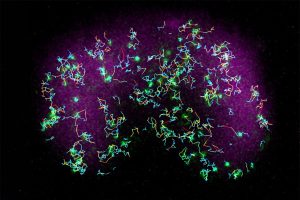
Scientists have long known that the cell membrane—the thin layer surrounding each cell—plays a crucial role in cell activities and communications. What hasn’t been clear, though, is how various proteins that are part of this membrane can dynamically change their location and grouping on the cell surface in response to various signals. Understanding this mechanism could not only provide a deeper comprehension of cellular dynamics in general but also pave the way for targeted medical interventions for diseases related to cell signaling and migration.
A team of researchers at the Johns Hopkins University, in collaboration with researchers from Osaka University and RIKEN Center for Biosystems Dynamics Research, Japan, have discovered a new method that selectively sorts certain proteins into distinct regions of cell membranes, providing insights into how cellular components organize themselves on a large scale during different physiological activities. The researchers introduced a mechanism called “dynamic partitioning,” which operates on the idea that specific proteins can naturally or in response to external signals gather or organize themselves on the cell membrane. This occurs by adjusting their movement in different areas of the membrane. “A dynamic partitioning mechanism polarizes membrane protein distribution” is published in Nature Communications.
“This discovery helps us to finally understand how proteins end up in different parts of the cell membrane and dynamically readjust their location as and when required. Our results provide a new lens through which we can understand how signaling events are tightly controlled, as cells communicate, migrate, or divide,” said research team leader Pablo A. Iglesias, the Edward J. Schaefer Professor of Electrical and Computer Engineering in the Whiting School of Engineering.
The team started the project after discovering that certain proteins, believed to be uniformly spread across the cell membrane, actually consistently undergo dynamic polarization during various cellular events. “We were intrigued by this surprising result and wanted to dig deeper,” said Tatsat Banerjee, lead author of the study and former PhD student in the Whiting School of Engineering’s Department of Chemical and Biomolecular Engineering and the School of Medicine’s Department of Cell Biology. “We speculated that we may learn some new membrane biophysics or cell biology here.”
To investigate further, Banerjee and his team used advanced microscopy techniques, including multimodal live-cell imaging, photoconversion, single-molecule imaging and optogenetics. These methods allowed them to observe and control events inside live Dictyostelium amoeba and mammalian immune cells in real-time, with detailed resolution at the subcellular level. When they combined computer simulations and real-life experimental results, they found that many proteins in cells don’t move because of vesicles (small, bubble-like structures that transport materials around the cell) or the cell’s internal scaffolding. Instead, these proteins move selectively based on the organization of the membrane and how specific proteins sense and interact with its spatial variations.
The researchers plan to investigate the biochemical reasons behind the uneven movement of membrane proteins. Additionally, they are keen on understanding the direct functional effects of these uneven distribution patterns. The study reveals an unexpected way that membrane proteins use to move and segregate in response to signals, without relying on the conventional energy-intensive biophysical mechanisms.
“We think that the mechanism described in the study could potentially be a fundamental guiding principle in biological pattern formation,” said Peter N Devreotes, the Isaac Morris and Lucille Elizabeth Hay Professor and Distinguished Service Professor in the Department of Cell Biology at the School of Medicine, who co-directed the study, “with the ability to advance the treatment of autoimmune disorders, heart diseases, memory issues, cancer metastasis, and various other pathological conditions associated with the dysregulation of cell signaling or movement.”
Other contributors include Debojyoti Biswas from the Whiting School of Engineering, with Yuchuan Miao and Dhiman Sankar Pal from the Johns Hopkins University School of Medicine. The collaborative effort also involved Satomi Matsuoka, Yoichiro Kamimura and Masahiro Ueda from the RIKEN Center for Biosystems Dynamics Research and the Graduate School of Frontier Biosciences at the Osaka University, Japan.
The study was funded by the National Institutes of Health, Defense Advanced Research Projects Agency and the Air Force Office of Scientific Research MURI. This work was also supported by funds from Japan Science and Technology Agency, Japan Agency for Medical Research and Development and JSPS KAKENHI.