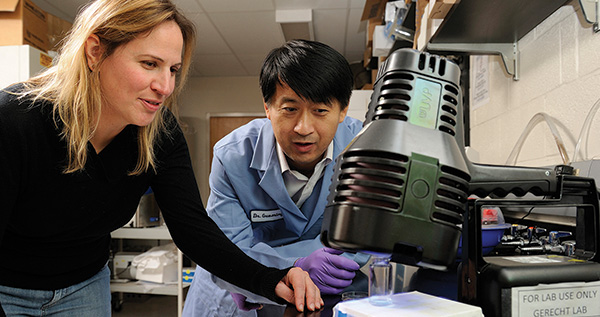
Sitting in a petri dish, hydrogel resembles a tiny jellyfish you might come across during a vacation walk along the ocean’s edge. It’s transparent, colorless, and odorless.
Smear it on third-degree burns in mice, however, and its true power is revealed: Within days, those wounds begin to heal. Three weeks later, recovery is so advanced that hair is sprouting on the surface of the rodents’ tender new skin.
“It’s definitely pretty amazing,” says Sharon Gerecht, an associate professor in the Department of Chemical and Biomolecular Engineering, and leader of the team that developed the material. (Clinicians from the Johns Hopkins Burn Center at Bayview Medical Center and the School of Medicine’s Department of Pathology were part of that team.) “We were frankly surprised that it worked so well.”
Though the simple substance comprising a water-based, threedimensional network of polymers has only been tested so far in laboratory mice (studies in pigs are about to begin), Gerecht and her team believe the gel has enormous potential not only for the treatment of human third-degree burns (which reportedly afflict more than 100,000 Americans annually) but also in healing diabetic foot ulcers and other wounds that happen when vital blood vessels carrying oxygen-rich blood have been seriously damaged or destroyed.
“We found that the inflammatory cells have a really easy time penetrating the gel, so that blood vessels are able to move in quickly and support the growth of new tissues there,” says Gerecht, who also is affiliated with the Institute for NanoBio- Technology. “This is exactly what we designed the gel to do, and what we hoped would happen.”
Interestingly enough, the researchers aren’t precisely sure how hydrogel works. Gerecht wonders whether it is somehow able to “recruit” stem cells, either circulating in the mice’s bloodstream or in the surrounding tissues, and signal them that they need to become new skin and blood vessels. It is also possible, she says, that the structure of the hydrogel directs the tissue repair in some way she and her team don’t yet understand.
One great advantage of Gerecht’s hydrogel is that it is purely synthetic and contains no pharmaceuticals or animal-derived ingredients, so the Food and Drug Administration (FDA) will likely consider it a Class II medical device. (Class II medical devices are considered “medium risk,” and include hearing aids and syringes.) Class II devices take less time to go from “laboratory to clinic” than do Class III devices, such as heart valves and pacemakers, which are considered “high risk,” according to Ian Tolfree, MS ’05, PhD ’09, a business analyst with Homewood Intellectual Property, which manages IP and technology commercialization for the Homewood campus.
In fact, Tolfree posits that if the upcoming pig studies turn out to be as promising as Gerecht expects, the hydrogel could be on the market for veterinary/animal use within six to 18 months. He expects that the gel will be valuable in hard-to-treat canine and feline wounds and skin conditions, as well as in slow-to-heal equine hoof and lower leg wounds, among other things.
“To get a human product on the market will take a bit longer, though not much,” says Tolfree, who notes that the university has filed patents to protect the invention and signed a licensing agreement with one local angel investor. “The main chemical ingredient here is a form of sugar currently used in bread, and which has been used clinically since the 1940s with a long safety record. I anticipate seeing hydrogel being used clinically in pre-approved trials on humans within 18 to 24 months.”
Gerecht and her team are also working on forms of hydrogel that are infused with various forms of human stem cells in an effort to trigger the body’s power to regenerate blood vessels. (Stem cells are undifferentiated or “blank” cells that have the potential to grow into almost any kind of tissue within the human body.)
“The idea is to harness what we know the body can already do, and enhance that ability with our biomaterials,” she says. “We know the biologies that are important for vasculature and are engineering them into our systems. There is still much work that must be done before we can make it work the way we want it to. But I think we are on the right track.”
Gerecht’s work is funded in part by the National Institutes of Health and the National Science Foundation.