Navigating via computer-based mathematical models, Pablo A. Iglesias aims to understand—and regulate—how cells move and divide.
Call him a control freak, but Pablo A. Iglesias covers more ground than you can imagine in his efforts to direct the movement of cells. Iglesias is steering his considerable knowledge of control engineering down a new path in cell biology that could well lead to extraordinary discoveries.
A professor in the Whiting School’s Department of Electrical and Computer Engineering, Iglesias has joint appointments in the departments of Biomedical Engineering and Applied Mathematics and Statistics. That’s a testament to his own flexibility in pursuing a path. The Venezuelan native earned his doctorate in control engineering at Cambridge University in 1991.
Control engineering is the study of automatic regulating systems, which often take the form of small devices silently managing mechanisms and household appliances. From thermostats to cruise controls, these control systems perform surprisingly sophisticated and dynamic tasks. But they’re nothing new. To make that point, Iglesias turns the clock back to 1903 and the Wright Brothers. “In fact, their greatest contribution to flight wasn’t the aircraft itself,” he notes, “but the control system that allowed them to fly the craft, which is control engineering.”
Today’s control engineers employ not bike chains and canvas but computer-based mathematical modeling systems in order to test-drive controllers in theory before they are actually engineered. Employing the principles of control theory, the control engineer studies and adjusts a mathematical model to create a predictable set of variable responses within a dynamic system.
Pedaling on the Biological Signaling Pathways
For Iglesias and his team at the Cellular Signaling and Control Laboratory, the challenge is applying this discipline to the study of biological signaling pathways, the amazingly complex regulatory system within the human body. “At the simplest level, it’s everything that happens inside a single cell that regulates its well-being,” says Iglesias. From body temperature to cholesterol counts, “No matter the scale, all of the body’s processes are very tightly regulated along much the same lines as apply to control engineering.”
First fascinated by this parallel seven years ago, Iglesias now focuses on two aspects of biological signaling pathways at the cellular level. The first investigation in his laboratory involves the study of chemotaxis, the movement of single cells, or even larger multicellular organisms, in response to the introduction of certain chemicals into their environment. For example, human white blood cells will pursue and destroy invasive bacteria, but only because a bacterium secretes a particular chemical detected by certain sensors on the cell.
In particular, Iglesias seeks to understand a human cell’s biochemical “guidance system” in terms of how it regulates chemotaxis. “Right now, we’re specifically investigating the possibility of feedback loops,” he says. “We have a mathematical model that shows that some of a cell’s observed behavior can be explained by these feedback loops, which measure an outside chemical concentration, produce a response, and then feed it back to the cell’s sensors.” The next step in this study, says Iglesias, will be to move from modeling a cell’s guidance mechanism to developing models of how the cell’s actual locomotion takes place.
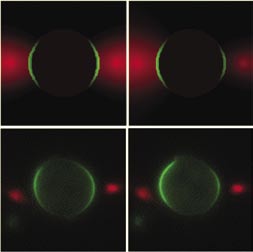
Inside a Cellular Split
In a second study, Iglesias and his team are investigating the control dynamics of cell division—and the steps that must occur for a cell to divide successfully. Following division, the resulting two daughter cells must each contain an equal half of the chromosomes from the original cell. However, if these genetic materials are not distributed equally, a condition called aneuploidy occurs. Iglesias notes that aneuploidy is one of the leading causes of genetic disease and cancer.
To gain a greater insight into the control mechanisms governing successful cell division, Iglesias and his colleagues are creating models to analyze cytokinesis, the final step in cellular division, where the two new daughter cells actually separate. To test for the presence of a feedback loop in the cell during this process, Iglesias collaborates with researchers at the Johns Hopkins School of Medicine’s Department of Cell Biology. Together they are developing an apparatus to be used during cell division—a dual micropipette aspirator that can apply pressure to cells through a microneedle less than five microns wide. “When a cell is dividing, we will actually apply some force to it that works against its division,” says Iglesias. “What we think is going to happen is that the cell will then push harder to divide itself. Just like a thermostat that works harder when a window is left open, the cell’s feedback mechanism will kick in to correct the discrepancy.”
Down the Road: Controlling Disease
All of this research, while purely theoretical, has the potential for providing substantial results. For example, malignant tumors in the human body spread through chemotaxis, as do certain other diseases. Short-circuiting the process of how these chemical cues work could effectively halt the progress of such diseases. And, as Iglesias points out, studying cell division shines the light on promising developments in fighting cancer. “A number of the cancer treatments currently being tried are attempting to disrupt cell division in cancer cells,” he says. “So understanding the control system of cell division actually may lead to some treatment.”
The approach Iglesias takes has earned high praise from his colleagues. “The work he is doing is simply fantastic,” says Gerard G. Meyer, professor and chair of Electrical and Computer Engineering. “The promise of his research is enormous, in terms of understanding those biological control mechanisms that govern the onset of disease—and its possible prevention.”
Typically, Iglesias’s response is modest. “I’m often asked why I look at cell biology problems if I’m in electrical engineering,” he says smiling. “My answer is that I find it interesting. The knowledge that I have in systems theory traditionally has not been used in biology. So there are a lot of open problems where I think I can make a contribution.”
For more on Pablo A. Iglesias’s laboratory, visit www.ece.jhu.edu
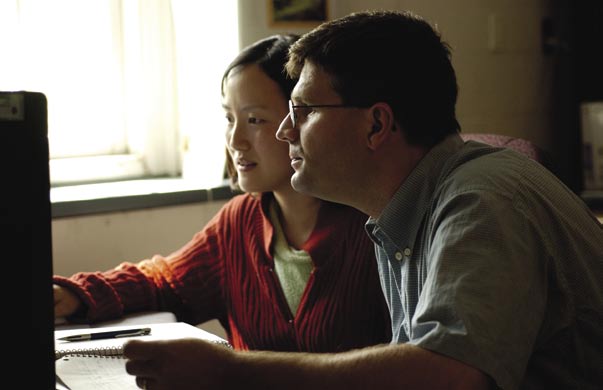