On the nano level, Evan Ma triggers structural changes to give copper and other metals exciting new twists in performance.
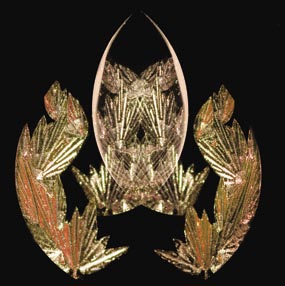
In modern materials science, more exotic materials like carbon nanotubes and biomaterials may sometimes upstage metals. But En “Evan” Ma has been getting a lot of attention lately for his breakthrough work with nanostructured metals. In fact, the Whiting School of Engineering professor of Materials Science and Engineering was recently honored with ASM International’s 2004 Materials Science Research Silver Medal “for important experimental and theoretical contributions in the study of metastable and nanocrystalline materials.” Ma, who earned his PhD at Tsinghua University in China with thesis work at the California Institute of Technology, is also affiliated with the Whiting School’s Center for Advanced Metallic and Ceramic Systems.
“We wanted to tailor the metal to achieve a great combination of strength and ductility.” En “Evan” Ma
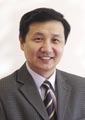
Putting it in the simplest terms, Ma sees himself “working in an old metallurgy field in which we try to discover something new.” Since the Bronze Age, advances in the use of metal have profoundly influenced human civilization. Our ancestors sought ways to gain advantage or solve problems by altering the characteristics of the metals they extracted from nature. Whether forging a blade sharp enough to penetrate the enemy’s armor, setting off precious jewels, supporting tall buildings, or containing tiny explosions inside an engine, humans extracted, fired, quenched, mixed, shaped, and otherwise changed metals to create new possibilities.
The difference today is the depth of our understanding of what’s going on in the structure of metals as they undergo these man-made changes. “When the electron microscope was invented,” says Ma, “scientists began actually seeing metallurgical microstructures on nanometer scale.” Researchers could see how those structures were altered by treatments that were already in use or being developed, and this enabled them to conceive of ways to use both existing and new metallurgical techniques to achieve new levels of performance.
Materials scientists now understand that it’s all a matter of equilibrium, and the lack thereof. Most engineering materials in use are somewhat unstable. Given enough time and temperature, they would approach thermodynamic equilibrium and stability. But new and often valuable properties of metals arise in states far from equilibrium. So this is where Ma takes them.
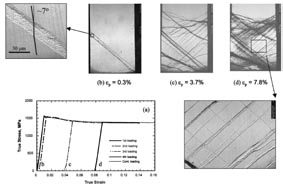
In his earliest studies, Ma used ion beam mixing. He accelerated ions of atoms and shot them into a matrix to trigger structural changes. In later years, Ma investigated self-propagating reactions of elements in multilayered geometry (see cover image, repeated above), and employed severe plastic deformation to mechanically force the formation of highly nonequilibrium materials. He also has used a vapor quenching technique to alloy metals that wouldn’t otherwise mix. That process involves heating two metals until they vaporize, then condensing the mixed atoms into a solid on a cold substrate. In a recent review published in Progress in Materials Science, Ma describes the metastable nature of the alloys created between these immiscible elements. The structure of the new alloy can be further evolved with heat treatments, yielding different performance characteristics along the way. Currently, Ma’s group is working on creating different structures in an amorphous (structureless) alloy.
Obviously, new scientific advances have made possible some unusual processing techniques and novel alloys. However, in a recent project, Ma and his colleagues also showed that it is possible to use strictly traditional tools to make a well-known metal behave in an unprecedented way.
Customized Copper
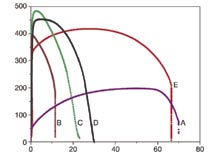
Copper is known to be a very ductile metal, easily stretched and suitable for making electrical wire. But copper is normally quite soft. Knowing that conventional copper is almost in equilibrium and has a structure of relatively large microcrystals, Ma set out to introduce smaller nanocrystalline grains into the copper microstructure because they tend to enhance strength. “We wanted to tailor the metal to achieve a great combination of strength and ductility,” he says.
Ma’s research team succeeded by finding the right combination and sequence of fairly conventional treatments. First, a 1 inch cube of copper is cooled to -200º C. Then it is run though hard rollers that flatten it out into a 1mm-thick sheet. The sheet is then heated to 200ºC. By controlling the cooling and heating steps, the proportion of nanoscale crystalline grains in the copper is adjusted. With optimized level of these small grains, the copper exhibits significantly higher strength—about six times the normal level—without sacrificing its high ductility. Easy to produce and endowed with a nice combination of performance characteristics (strength and ductility), such a new form of metal is attractive for a range of applications. Similar customization of nanocrystalline metals makes it possible to customize metal parts for micro machines known as microelectromechanical systems, or MEMS.
Tailor-made Tungsten

Ma also is collaborating with his colleagues in Mechanical Engineering to develop anti-armor materials for use by the military. For years, armor-piercing projectiles have been made with depleted uranium. This high-density material exhibits a highly desirable self-sharpening capability. Rather than mushrooming on impact, it spirals and maintains a pointed shape that penetrates armor plating. However, the use of uranium is controversial, so the search is on for an alternative. Ma thinks he and his colleagues may have the answer.
Tungsten, like uranium, is a very strong, very dense material, and it has a high melting point. But it lacks the critical self-sharpening capability. However, by pushing the tungsten to extreme nonequilibrium and carefully controlling the resulting nanostructured grains, Ma’s team has already been able to achieve shear localization in the metal. The plastic flow concentrates in a localized shear zone, so the material shears off at a 45º angle—potentially useful as a self-sharpening penetrator material.
With the freshly minted tools of nanotechnology at their command, materials scientists like Evan Ma are truly the alchemists of the new millennium.
To learn more about Evan Ma’s research, visit www.jhu.edu/~matsci/people/faculty/ma/ma.html