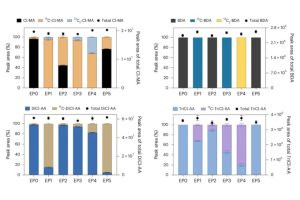
Scientists have known for decades that chlorination of drinking water creates unwanted disinfection byproducts but haven’t fully understood how these toxic compounds form. New research by scientists at Johns Hopkins, Towson University, and the University of Southern California has revealed how that process happens, a vital step in solving the problem.
The findings, “Stable isotope labelling to elucidate ring cleavage mechanisms of disinfection by-product formation during chlorination of phenols,” were published in the journal Nature Water.
The problem of disinfection byproducts, or DBPs, is ubiquitous. Ninety percent of water treatment systems in the U.S. use chlorine to disinfect drinking water. And phenols – chemical compounds that occur naturally in the environment and are abundant in personal care products and pharmaceuticals – are commonly found in untreated water. Scientists know that when chlorine reacts with phenols in water, it can create these toxic byproducts. Some DBPs, such as chloroacetic acids and trihalomethanes, are regulated in drinking water because of their potential health risks. Others are less well understood but may also be harmful.
“Finding a balance between the elimination of pathogens and the unintentional formation of DBPs is very challenging. Understanding the role of specific compounds that contribute to the formation of DBPs together with the underlying chemical reaction mechanisms can help us to improve the design of drinking water treatment systems,” said Carsten Prasse, associate professor in Johns Hopkins’ Department of Environmental Health and Engineering and corresponding author on the study.
To crack the puzzle of how these byproducts are formed, researchers used specially labeled molecules containing a rare form of carbon called carbon-13 to track exactly how phenols change into DBPs during chlorination. They discovered four main chemical pathways that break down the ring-shaped phenol molecules into smaller, potentially harmful compounds. Three of these pathways involve the carbon atoms from the phenol’s ring structure, while the fourth pathway involves a carbon atom from a side group attached to the ring.
The findings provide clear evidence of distinct chemical processes that form several important DBPs, including both well-known and newly recognized ones. By showing that certain byproducts form through unique pathways, the research confirms previous guesses and offers a deeper understanding of how these harmful substances are created.
Understanding these reaction pathways helps pinpoint which starting materials lead to these toxic chemicals in drinking water. Knowing what triggers the formation of these DBPs can help water treatment facilities adjust their processes to reduce the levels of dangerous compounds.
“This research represents a significant step forward in understanding how water disinfection processes can inadvertently create toxic compounds, which could ultimately lead to safer drinking water treatment methods,” said Prasse. “We’ve unlocked the door to research that can expand testing beyond currently regulated DBPs, develop better methods for detecting hard-to-measure DBPs, and continue using isotope-labeled molecules to study how other water treatment processes might create unexpected byproducts.”
Prasse suggests the next step for researchers is to look at intermediate compounds—like certain chlorinated benzoquinones—that might be key stepping stones to creating harmful DBPs.
“By doing so, we can refine how we control DBP formation, improve the safety of drinking water, and ultimately protect human health,” he said.