They say that beauty lies in the eye of the beholder. But sometimes it’s too small, too complex, or even too fast for the naked eye to comprehend. Using advanced technology, our engineers are making the unseen visible—and transforming science into art.
Tiny Tats
David Gracias
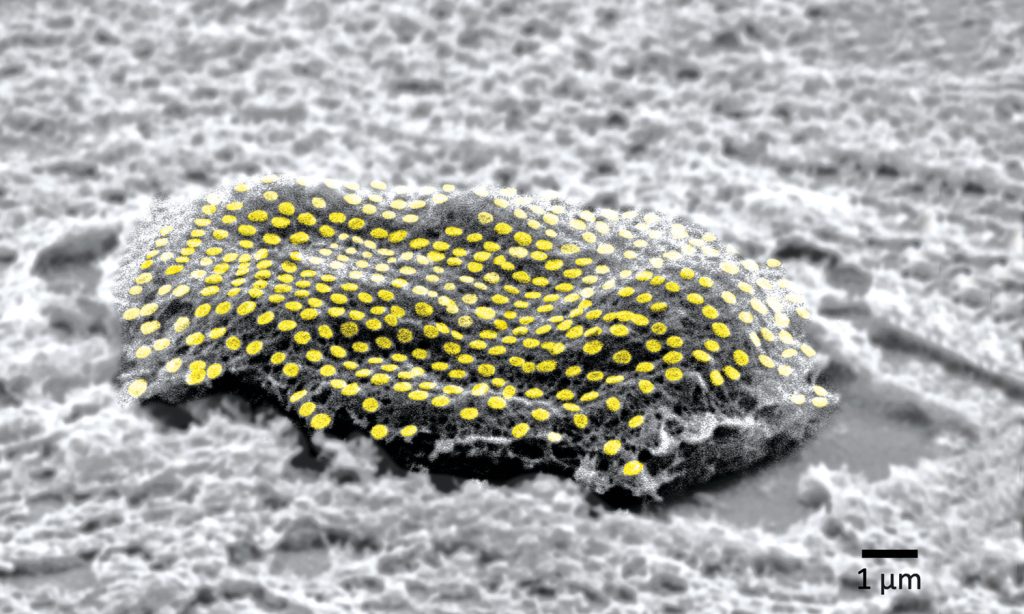
These gold dots, hundreds of times smaller than the head of a pin, may signal the future of disease diagnosis and treatment. Developed by David Gracias, a professor of chemical and biomolecular engineering, the dots are lithographically patterned tattoos that contain model patterns of optical elements and electronics and can be stuck to individual live cells using a molecular glue. Gracias’ goal is to be able to remotely track the health of isolated cells using biosensor technologies. “We would like to have sensors to remotely monitor and control the state of individual cells and the environment surrounding those cells in real time,” Gracias says. “If we had technologies to track the health of isolated cells, we could maybe diagnose and treat diseases much earlier and not wait until the entire organ is damaged.”
BIRDS OF A FEATHER
Jochen Mueller
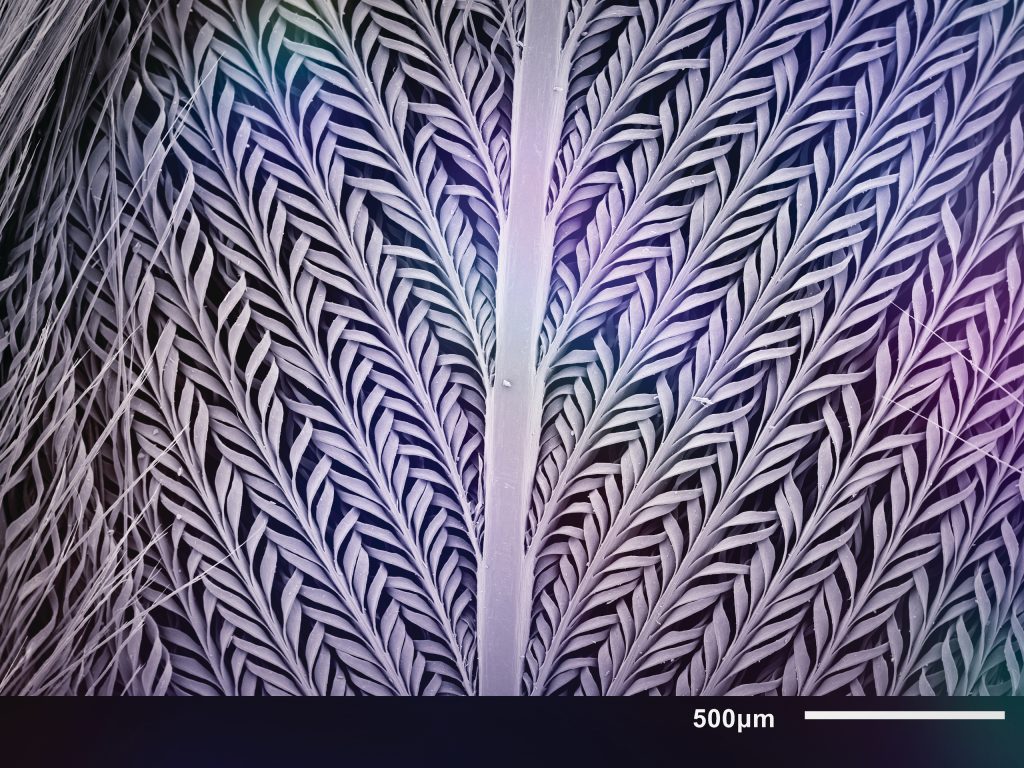
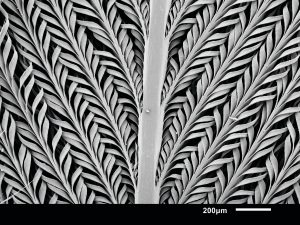
Using high-resolution microscopes and 3D technology, Jochen Mueller, an assistant professor of civil and systems engineering, and researchers at Massachusetts Institute of Technology, have captured an unprecedented view of feathers from a male desert-dwelling sandgrouse, a bird known for its ability to carry water—up to 15% of its body weight—in its belly feathers. High-tech imaging technologies enabled the team to examine the feathers’ microstructure. Looking closely at the shafts, each just a fraction of the width of a human hair, and the even tinier individual barbules, they identified components in the feathers’ structure that are optimized to hold and retain water. “That’s what excited us, to see that level of detail,” Mueller says. This knowledge could help inform the design of new materials for absorbing, retaining, and releasing liquids.
DEALING WITH REJECTION
Joshua Doloff
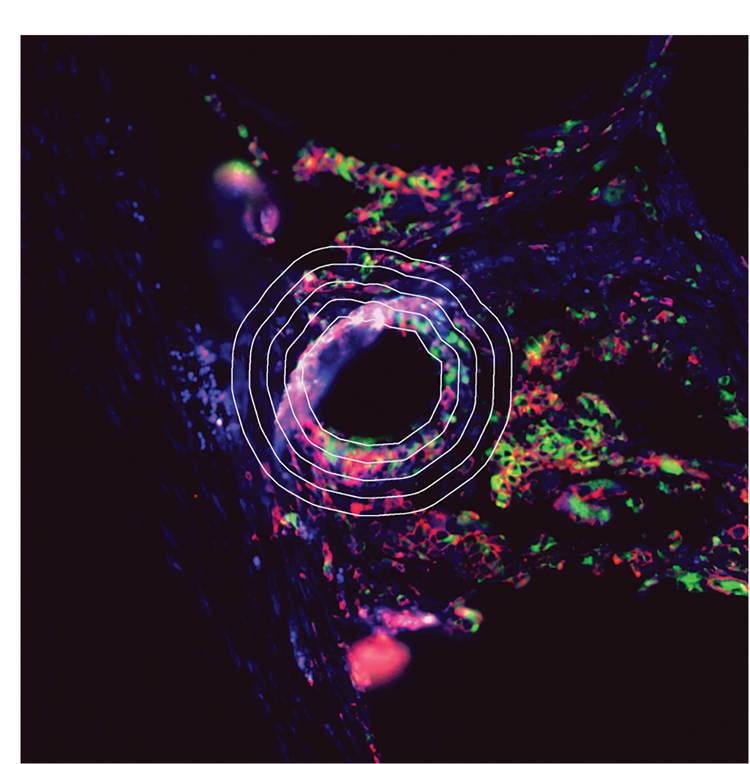
These swirls of bright colors, images of tissue stained with fluorescent labels, may herald a breakthrough in preventing medical implant rejection. Joshua Doloff, an assistant professor of biomedical engineering, discovered an advanced “humanized” mouse model that he is using to investigate why scar tissue forms around an implanted device, a process known as fibrosis. Doloff and his team inserted different material implants in the genetically modified mice to see how and where immune cells would gather around the implants over time. Using fluorescent microscopy to view tissues that had been stained beforehand with fluorescent labels, the researchers were able to use a multiplexed antibody array to detect the location (as a function of distance away from the implant surface) of human immune cell players, revealing events in the early development and progression of fibrosis.
CAN YOU SEE ME NOW?
Vishal Patel and Nithin Gopalakrishnan Nair

One obstacle to ensuring the accuracy of facial verification is that the software simply doesn’t work well in low-light or nighttime conditions when images must be taken using thermal cameras. But Vishal Patel, an associate professor of electrical and computer engineering, and Nithin Gopalakrishnan Nair, a PhD student in electrical and computer engineering, have devised a way to improve facial recognition in these challenging situations using their denoising diffusion probabilistic model, which can convert “noisy” images created with thermal cameras into clear, photo-like ones. Using a diffusion process to train their model, they showed the system pairs of images—one, a normal photo, and the other, a thermal image of the same scene. By comparing the images, the system learned to understand the “relationships” between regular photos and thermal images, learning how features such as faces can be transformed from the thermal to the visible domain.
SHINE BRIGHT LIKE A NANODIAMOND
Ishan Barman
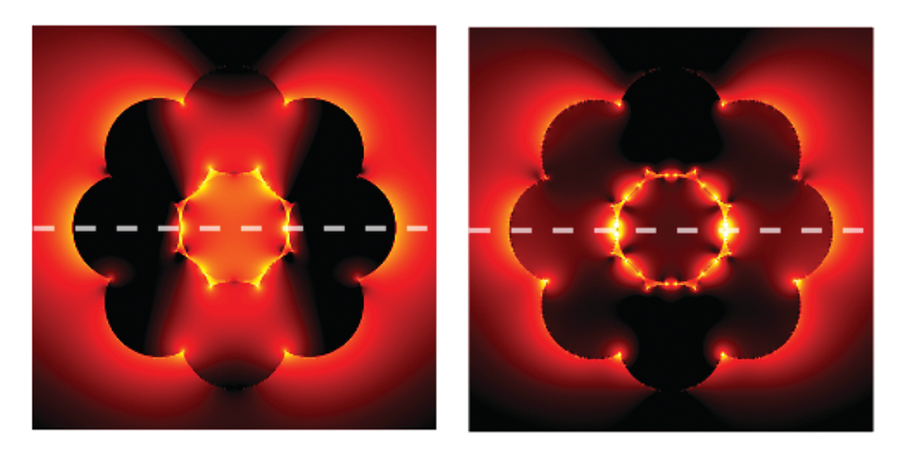
Jewelers consider cut, color, clarity, and carats when evaluating a diamond’s worth. But in the quantum world, another quality is more valuable: the presence of nitrogen-vacancy (NV) centers. These light-emitting defects in the jewels’ lattice are potent sources of quantum particles that have the potential to be used in sensing applications ranging from biological imaging to electrical and field sensing and more. Until now, it has been challenging to attach NV centers with structures that amplify their optical properties. Ishan Barman, an associate professor of mechanical engineering, has come up with an original solution. He is pairing NV centers with DNA’s self-assembling abilities to create high-performance hybrid plasmonic nanodiamonds: infinitesimal diamond-like particles that can control light in unique ways. The innovative approach resulted in the components arranging themselves into a closed nanocavity that surrounded the nanodiamond: an arrangement with a profound impact on the nanodiamond’s brightness and emission rates.
HAVING A BALL WITH OPTIMIZATION
Benjamin Grimmer
Benjamin Grimmer designs and analyzes optimization algorithms—a field that is frustratingly abstract to many. So the assistant professor of applied mathematics and statistics is using a 3D printer to turn these complex concepts into colorful, tangible objects that let students explore decisionmaking across 1D, 2D, and 3D dimensions. Each ball serves as a visual indicator of an operation’s strength: its maximum possible efficiency in accomplishing specific tasks or outcomes. The colors denote the problem’s level of difficulty: red (challenging) and green (easy). For the golden balls with white bases, the problems’ difficulty is an open question. “Real-world optimization problems can have hundreds or thousands of variables, so students really need to use their mathematical brains to extrapolate how to think about them. 3D examples give them one more stepping stone before they have to jump off to work in mathematical generalizations,” Grimmer says.