The molecular switches being created in Marc Ostermeier’s lab could one day target chemotherapy or sound a warning about anthrax.
It sounds like the premise for the next blockbuster monster movie. Ensconced in his laboratory, a gifted young scientist employs the power of evolution to create new forms of biological material that will do his bidding. However, in this case, the story’s true. And it all hinges on a single class of molecules called proteins.
Over the past 20 years, science has become increasingly interested in the possibilities raised by protein engineering, that is, genetically modifying proteins in order to create new and improved versions. Such engineered proteins can be tailor-made to support an array of new applications in medicine, agriculture, and industry. While protein engineering is still in its infancy, researchers like Marc Ostermeier are well on their way to making it the stuff of everyday life. For Ostermeier, assistant professor in the Department of Chemical and Biomolecular Engineering, the protein is a tiny gateway to enormous possibilities.
By coupling two proteins to create a molecular switch, the Ostermeier Lab is investigating specialized molecules, microscopic partnerships in which one protein controls the activity of the other. Among their applications, Ostermeier cites such possibilities as a protein that, sprayed on any surface, would instantly fluoresce when it detects a certain biological agent like anthrax. Molecular switches could also prove to be a boon in diagnostic testing by revealing the presence of a certain type of cell or virus. In targeted drug delivery, a molecular switch could serve as a carrier for an injected chemotherapeutic drug, not releasing it until it senses a cancer cell, and thus allowing larger and more effective chemotherapeutic doses to be given.
Finding the Best Among Millions
In terms of biological processes, proteins are fascinating and essential components. Composed of chains of amino acids arranged in specific sequences, proteins perform vital activities in the functionality, structure, and regulation of the human body. What’s more, a range of different proteins exist—including enzymes, collagen, antibodies, and hormones—each of which is uniquely dedicated to particular biochemical tasks.
Ostermeier, who joined the Whiting School in 2000, and his lab colleagues have managed their research in protein engineering through a process called directed evolution. As he explains, “In Darwinian evolution, you have variation of a species, and then some selective pressure is applied so that only the strongest survive. In the same way, we’re trying to use Nature’s algorithm in the lab to evolve proteins with the properties we want. We make millions of variations of a protein in a single test tube, and then evaluate them all at once, selecting only those that are improved in the properties we want.”
“We need to be able to control when proteins do their job.” Marc Ostermeier
Employing this accelerated process of evolution, Ostermeier and his graduate assistant, Gurkan Guntas, have focused on a particular area of improvement generally overlooked by protein engineers: controlling a protein’s function. In the human body, some proteins naturally have this ability, having functionally evolved with these properties. “We need to be able to control when proteins do their job,” Ostermeier notes. “We have to be able to turn them on and off. So I was thinking about how could we engineer this control—to tell a protein to turn on its function when a certain condition existed. That’s how I got into trying to make a molecular switch.”
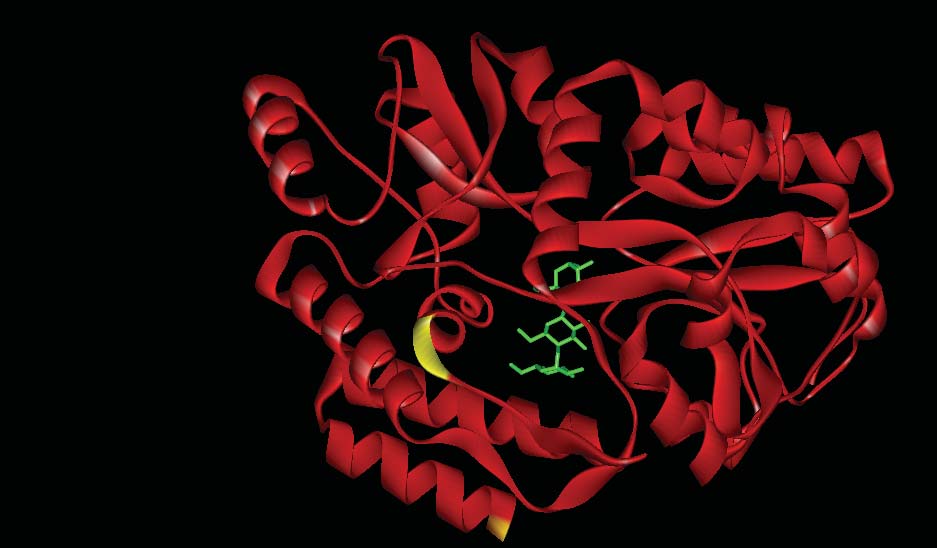
On the Way to Useful Biological Agents
To engineer such a molecular switch, Ostermeier first determined that he needed two proteins—one with the function that needs to be controlled, and the second with the ability to recognize the signal to turn it on. By genetically splicing two complementary proteins together through a technique he calls domain insertion, Ostermeier would create a fusion protein that modulated its activity and generated certain effects when in the presence of specific outside conditions or agents. Then, using the directed evolution process, he would refine this new protein to increase its effect—in this case, the difference in function between the on state and the off state.
To demonstrate that his approach could be accomplished using any two proteins, Ostermeier deliberately chose two that had nothing in common to build his first switch. One of the proteins, beta-lactamase, is an enzyme that can disable penicillin-like antibiotics. The other is the maltose binding protein found in a harmless form of E. coli bacteria; it binds to a type of sugar called maltose that the E. coli cells can use as food.
After creating a fusion protein from the two and subjecting it to directed evolution, the researchers ultimately were able to find several fusion protein variants in which both original proteins were still active, and more importantly, in which the presence of maltose actually caused the beta-lactamase partner to step up its attack on an antibiotic.
“In other words,” Ostermeier recalls, “one part of this coupled protein sent a signal, telling the other part to change its behavior. This is the first clear demonstration that you can apply the domain insertion technique to control the activity of an enzyme. If we can replicate this with other proteins, we can create biological agents that don’t exist in nature but can be very useful in important applications.”
Patents Pending, and a Buzz
Given the impact of Ostermeier’s discovery, buzz was sure to follow. On March 27, 2003, he presented his findings to the 225th annual meeting of the American Chemical Society in New Orleans. This winter, Guntas and Ostermeier published a paper documenting one aspect of the process in the February 6 Journal of Molecular Biology. The Johns Hopkins University has applied for U.S. and international patents related to Ostermeier’s molecular switch technology and the techniques used to produce them. In addition, building on his earlier grants from the American Cancer Society and the Maryland Cigarette Restitution Fund, the National Institutes of Health has awarded Ostermeier a five-year grant to continue his research.
As for Ostermeier, he sees no end to the opportunities in his research. Protein lengths may vary from as small as 40 amino acids to thousands or more. “In every protein that is 200 amino acids long, there are 20 possible variations in each of the 200 amino acid locations in the entire sequence,” he says. “So the possible number of different proteins you can make would be 20 to the 200th power—an extremely large number. By comparison, the total number of elementary particles in the whole universe is estimated to be 10 to the 79th power. So, even if I make a billion variations of a protein, I’m only creating an infinitesimally small amount of what can possibly be created.”
Visit the Ostermeier Lab at www.jhu.edu/~cheme/ostermeier
Special thanks to Phil Sneiderman for his contributions to this article.