In layers one atom thin, Jonah Erlebacher creates far more surface for catalysis, even for inert metals.
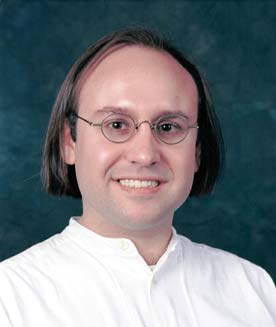
Jonah Erlebacher is not an alchemist, but he does work with gold—at the nanometer level. His goal is to further both its applications and its value to engineers and scientists. A nanoporous gold could be coated with enzymes and used in a biomedical device, for example. Or a metal could be fabricated for a specific purpose, perhaps to resist the corrosion that leads to mechanical failure in aviation. “I’m interested in materials that are macroscopic and useable in real technology, but also have some aspect of their structure that possesses features with nanometer scale,” says Erlebacher, assistant professor of Materials Science and Engineering.
Erlebacher and his group have developed methods to turn pieces of gold into a sponge-like porous metal, with pores that are less than 10 nanometers across (10 one-billionths of a meter). Their reasons for studying nanoporous gold are compelling: While gold is not a good catalyst because it is inert, nanoporous gold has one of the highest surface areas known of any metal. It has many unique chemical, mechanical, and electrical properties. And it has important applications in a wide variety of fields. Yet it has remained largely unstudied.
To learn more about nanoporous gold, Erlebacher begins with an alloy of silver and gold (12 carat white gold) that gets “dealloyed” by being dipped into nitric acid. The acid dissolves the silver and leaves behind an intricate three-dimensional nanoporous architecture. This spongelike warren is far different than the crystalline form so familiar to jewelers. Erlebacher works with this dealloyed structure as a building block for making new kinds of materials. The atoms then grow on the backbone of the porous structure, with a very important result: a much larger surface area than nonporous materials.
The ancient Incans knew the secret of stretching their supply of gold by mixing it with copper and then dissolving the alloy with salty substances to create a surface easily polished. But exactly why such dealloying worked had long been a mystery in materials science. In the March 22, 2001, journal Nature, Erlebacher and co-authors published their mathematical equations describing the evolution of porous gold, as well as their theory about why dealloyed gold atoms gather in rough, porous clusters. Simply stated, the gold atoms were acting like water droplets.
“In the past 10-15 years, there’s been a huge interest and activity in learning how to chemically tether useful, interesting species to gold surfaces,” says Erlebacher. With this in mind, he is studying issues such as what factors control the pore size, and how to coat the surface of the pores with catalytic and chemically functional compounds and elements.
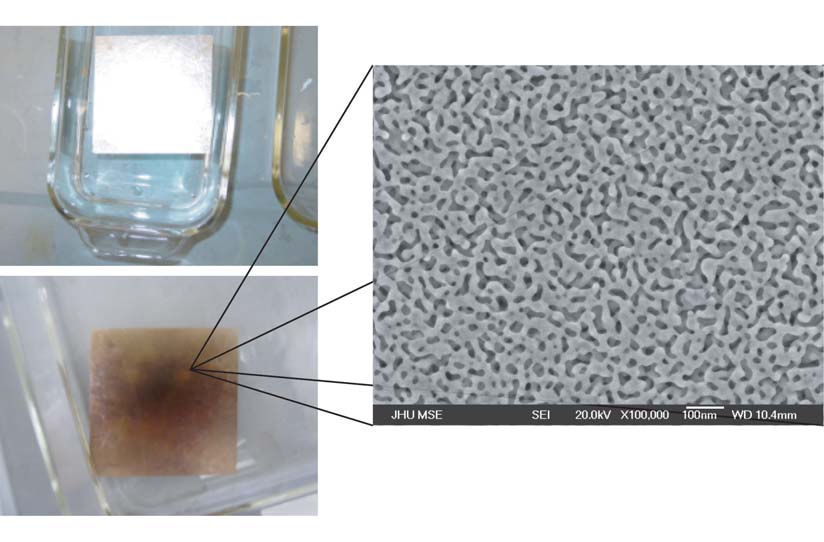
More Efficient Fuel Cells, and Artificial Cell Membranes
One application would be to improve upon current fuel cell technology, which Yi Ding, one of Erlebacher’s graduate students, is now studying. Says Erlebacher, “Fuel cells rely heavily on the ability to make catalyst materials with an extremely high surface area. Central to fuel cell and many other catalytic technologies, like catalytic converters in your car, is the unfortunate fact that the best catalyst in the world—platinum—is also extremely expensive. So, you have to figure out a very efficient way to use all the catalyst material.”
Erlebacher and Ding have recently done just that, developing a way to cover the gold with just a one-atom thick layer of platinum. With this structure, every atom is available for catalysis. Working with the Hopkins Office of Licensing and Technology Development, Erlebacher and Ding have two patents pending on this technology.
But Erlebacher isn’t stopping there. He is also working on how nanoporous gold can be useful in biotechnology, collaborating with an interdisciplinary research team that includes professor Michael E. Paulaitis and research professor Mathias Lösche, both in Chemical and Biomolecular Engineering; and John Kasianowicz at the National Institute of Standards and Technology. By using nanoporous gold as a substrate to which is tethered an artificial cell membrane, the team hopes to use porous gold membranes to greatly enhance the study of the structure and function of cell membrane proteins. The team recently received funding from the National Science Foundation to pursue applications such as this one in biotechnology.
When it comes to working with the sometimes odd characteristics of nanomaterials, Erlebacher’s favorite mantra is, “It’s not a bug, it’s a feature.” With nanoporous gold, these features are also opportunities for advancements in nanotechnology, biotechnology, and materials science.
Visit Jonah Erlebacher’s group at www.materials.jhu.edu