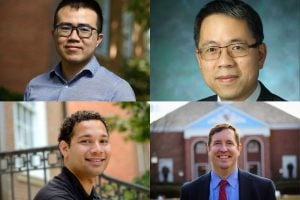
Four Department of Materials Science and Engineering faculty are among the 148 Johns Hopkins affiliates to earn 2024 Discovery Awards. Launched in 2015, these awards aim to bring faculty together from across the institution to solve complex problems in innovative ways. Proposals selected for 2024 center around themes of health care improvement, climate change, and artificial intelligence (AI).
Michael Kessler Louis M. Sardella Professor and department head; Hai-Quan Mao, professor and INBT director; Dingchang Lin, assistant professor; and A. Shoji Hall, assistant professor, will team up with colleagues from the Whiting School of Engineering, the Bloomberg School of Public Health, the School of Medicine, and the Krieger School of Arts and Sciences on the following projects:
Title: Novel Materials for Custom-Tuned Earplugs for Musicians: A Multifaceted Approach
Principal Investigators: Michael Kessler (Engineering), Kris Chesky (Peabody/Medicine) & Gurumurthy Ramachandran (Public Health)
Summary: The funded project “Novel Materials for Custom-Tuned Earplugs for Musicians” aims to address noise-induced hearing damage, a significant risk for musicians, by developing customized earplugs that adapt to individual needs and specific environmental conditions. The research involves a multidisciplinary team from four different schools at Johns Hopkins University. The approach includes evaluating different material systems, such as bio-based polymer blends, liquid crystalline elastomers, and nanocomposite foams, to create earplugs that provide effective hearing protection while preserving sound fidelity. These earplugs will be passively tuned using acoustic filters or dampers to achieve desired attenuation characteristics across different frequencies. The project will use dynamic mechanical analysis to measure the materials’ viscoelastic properties and create master curves for their damping behavior. The goal is to improve the long-term health and career sustainability of musicians by providing better hearing protection that does not compromise their ability to perform.
Title: Spatial mapping of the cortico-striatal-basal ganglia circuit using flexible nonlinear neural probes
Principal Investigators: Dingchang Lin (Engineering) & Patricia Janak (Arts & Sciences)
Summary: The basal ganglia, a network of brain regions, is important for motor planning, decision-making, and cognitive flexibility. The dorsomedial striatum (DMS) is a key part of this network, connecting inputs from the cortex and thalamus to the basal ganglia. To understand this network, we need to study the neural activity in the DMS, globus pallidus externus (GPe), and substantia nigra reticulata (SNr), along with their cortical and thalamic inputs. This has been difficult because traditional recording methods use linear probes, while brain circuits are non-linear. Linear probes can only record from one brain region at a time. The team plans to develop a method to implant flexible probes along brain circuits with minimal surgery. These probes will be used to study neural activity in rats during decision-making and can record for months to over a year, allowing long-term study of neural circuits during different stages of learning.
Title: Brain-wide mapping of astrocyte activity using genetically encoded crystal recorders (GECRs)
Principal Investigators: Dwight Bergles (Medicine) & Dingchang Lin (Engineering)
Summary: Astrocytes are an essential component of neural circuits. They form highly interconnected networks, in which individuals occupy distinct domains that are extensively coupled through gap junctions. Calcium signaling is instrumental for astrocytes and has been linked to diverse phenomena, including changes in blood vessel diameter, synaptic plasticity, and neuromodulation. Nevertheless, knowledge about the role of calcium signaling in astrocytes in vivo remains rudimentary, due to lack of quantitative information about the interaction of astrocytes with distinct neural circuits and the behavioral contexts in which astrocyte networks are activated. In this work, the team will craft an intracellular recording platform that specifically targets astrocytes in vivo and temporally resolves their calcium dynamics across large brain regions. The team will leverage the intracellular recording platform pioneered by the Lin Lab to achieve the goal.
Title: Programmed Regenerative Inflammation for Functional Recovery in Repairing Volumetric Muscle Loss
Principal Investigators: Laszlo Nagy (Medicine), Hai-Quan Mao (Engineering) & Warren Greyson (Medicine)
Summary: Volumetric muscle loss (VML) presents significant challenges in regenerative medicine, requiring new solutions. This proposal combines efforts from three research groups to test the effectiveness of programmable composite scaffolds. These scaffolds will incorporate newly discovered factors that aid in muscle regeneration and modulate the immune response. High-dimensional transcriptomic analysis identified GDF-15, GDF-3, IGF-1, and osteoactivin (OA) as important for muscle regeneration, secreted by a new type of muscle-infiltrating macrophages called Growth Factor-Expressing Macrophages (GFEMs). The study aims to create implantable hydrogel fiber scaffolds that promote muscle repair by providing a supportive environment with regenerative inflammation. These scaffolds will deliver bioactive cues from GFEMs using nanoparticles infused in the hydrogel fibers. This method aims to enhance muscle regeneration and improve tissue repair and functional recovery. The innovative aspect is mimicking the healing properties of GFEMs to create an immune response that accelerates recovery from VML injury.
Title: Harnessing Single-Atom Plasmonic Catalysis for High-Efficiency Energy Transformations
Principal Investigators: Anthony Shoji Hall (Engineering) & Thomas Kempa (Arts & Sciences)
Summary: Reducing the energy intensity of industrial chemical processes is a vital component of accomplishing broader sustainability goals. This Discovery Award studies the photo-electrochemical production and decomposition of carbon-free hydrogen carriers, aiming to surpass the efficiency of current thermal processes. We hypothesize that plasmons (collective oscillations of mobile electrons) can guide reactions toward more efficient and desirable pathways, enhancing catalytic performance. Our approach has two main objectives: first, using 2D structures to study the impact of single-atom sites on catalytic performance without plasmonic effects; second, combining these structures with light to explore how surface plasmons influence energy transfer and catalytic activity. Using advanced electro-analytical techniques, we aim to uncover the mechanisms of single-atom catalysis and develop guidelines for designing better catalysts. Our work could lead to significant advancements in renewable energy technologies.
*Co-Funded with the Ralph O’Connor Sustainable Energy Institute*