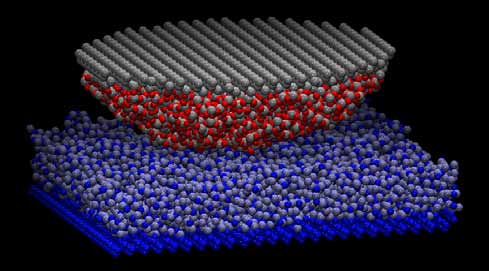
When it comes to technology, smaller is better. And as iPods shrink to the size of postage stamps, there’s a need to understand how different materials such as metals and silicon behave and react on a tiny scale. That’s where Michael Falk comes in. The associate professor of materials science and engineering uses computer simulation to examine materials behaviors on the nanometer scale—as tiny as 1/100th of the radius of a needle’s tip.
Using a simplified mathematical model of the material, a computer simulates what is going on inside a material as it performs its function, undergoes some stress, or is being processed into its final form. The simulation provides a moment-by-moment look at what happens to the atoms on a microscopic level during a reaction. This allows engineers to predict how a material is going to behave and change over time.
One of Falk’s projects is looking at structural applications for a group of materials called “metallic glasses.” These materials might be used to make precision medical instruments, for example. Unlike other metals, their atoms are not arranged in an ordered way. “There’s not a lot understood about why they behave the way they do, and the fact that they’re glasses as opposed to crystals makes it harder to interrogate their structure experimentally,” says Falk. “So the simulations have been something of a look into the structure of these disordered materials even though our experimental techniques are limited.”
Other projects of Falk’s include simulations of an atomic force microscope to understand friction in an oxidized semiconductor material on the nanometer scale, and simulations involving lithium ion batteries. “You can apply this modeling to a whole range of different kinds of materials,” says Falk. “We just have to come up with a mathematical description of the material and then we can apply some of the same techniques whether we’re looking at an atomic force microscope, or a metal being pulled, or at some reaction.”
Falk is particularly interested in extending molecular dynamics simulation to longer time scales. Currently, such simulations are limited to times shorter than a microsecond. “So if you wanted to look at something that took a millisecond, you’re out of luck,” says Falk. He and his students are working on techniques to look at slower processes.
In Falk’s view, theory, simulation, and experimental work go hand in hand. The model helps generate the question, so experiments can provide the answer. “The idea is if we can do these simulations and they show something interesting, then we can come up with a theory that gives the experimentalist some jumping-off point.”