
Water, wind, and riverbeds! Deep in the bowels of the Whiting School’s buildings, engineering faculty have replicated real-world physical environments—oceans, skies, streams—in mammoth experimental laboratories that will (in some cases, quite literally) take your breath away. We bring you a sampling.
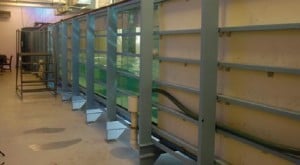
Surf’s Up! The 20,000-gallon wave tank in the basement of the Stieff Building is equipped with four hydraulic piston-actuated paddles that can create waves moving at different angles and frequencies—generating “a fairly realistic sea state,” says Tony Dalrymple, the Willard and Lillian Hackerman Professor of Civil Engineering. Sensors along the sides of the 58-footlong tank measure wave height and velocity. Dalrymple and his team develop algorithms to predict the behavior of waves and their impact on shorelines, then use the tank to field test their predictions—knowledge vital for preparing for hurricanes, and forecasting how beachfront construction projects will affect shoreline erosion over the long term.
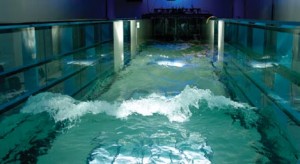
The pristine waters of the tank (the only one of its design in the nation) will soon get murkier. This spring, some 7 tons of muddy clay will be deposited over the tank’s bottom as part of a five-year, multi-university study that Dalrymple will lead. Fieldwork off the coast of Louisiana and elsewhere has shown that wave energy dissipates (a phenomenon known as “damping”) when waves travel over mud. How and why? Dalrymple hopes the Stieff Building’s ocean-in-miniature will hold some answers. “It’s not easy to see mud in the field,” he says, chuckling. “It’s kind of muddy.”
No Stone Unturned

The colorful stones arrayed along the bottom of the Erosion and Sedimentation Laboratory Tank create a deceivingly idyllic picture. Just wait until graduate student Brendan DeTemple sends water coursing down the flume, more than 1,000 gallons per minute. Then the stones and sand go tumbling down the 50-foot length. The sediment is removed and the stones get caught in the trap at the end (what DeTemple calls the “big blue bathtub”), where they are weighed, counted, and sorted. “We can collect more than 10 gallons of sediment in two minutes—it all depends on how we set up the experiment,” says DeTemple, a member of the Stieff Building lab led by Peter Wilcock, professor of geography and environmental engineering.
Measuring the rate at which the sediment is transported, and the composition of the transport (the percentage of sand vs. gravel), can help the engineers better predict how riverbeds will respond to floods, and how a river will evolve over time. “How long will a reservoir last? That depends on how much sediment is coming in,” says DeTemple. “Eventually, all reservoirs will fill up with sediment.”
There are implications for wildlife, too, he notes. “Salmon will spawn in gravel but not sand.”
In the Flow

Research scientist Hyung-Suk Kang finishes adjusting the anemometer system in the Corrsin Wind Tunnel, then quickly ducks out before the air stream is activated—at speeds up to 50 miles per hour. Relatively speaking, however, that’s not all that fast. “This is known as a low-speed wind tunnel. While there are wind tunnels that go at supersonic speeds, this one produces very high-quality flow,” explains Charles Meneveau, the Louis M. Sardella Professor of Mechanical Engineering.
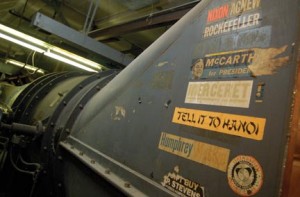
The closed-loop tunnel in Maryland Hall has a rich history stretching back to the early 1960s, a history emblemized by the vintage bumper stickers that still adorn its secondstory metal fan shaft. Designed and built during the golden years of fluid mechanics at Hopkins by the late professor Stanley Corrsin, the wind tunnel in Maryland Hall has over the years provided data for landmark papers in the field of turbulence. Today, Meneveau and his colleagues use the Corrsin Wind Tunnel to conduct tests on objects placed in the 10-meter-long test section and draw conclusions about the behavior of airflow and heat-flow. Such information is crucial to understanding turbulent air flow around automobiles and aircraft, ventilation inside buildings, and atmospheric wind over plant canopies and rough terrain. It’s exceedingly precise work. Says Meneveau, “The turbulence that we study in there reaches down to very small eddies, fractions of millimeters, measured at 40 kilohertz—which means that 40,000 times a second there’s a velocity measurement being done.”
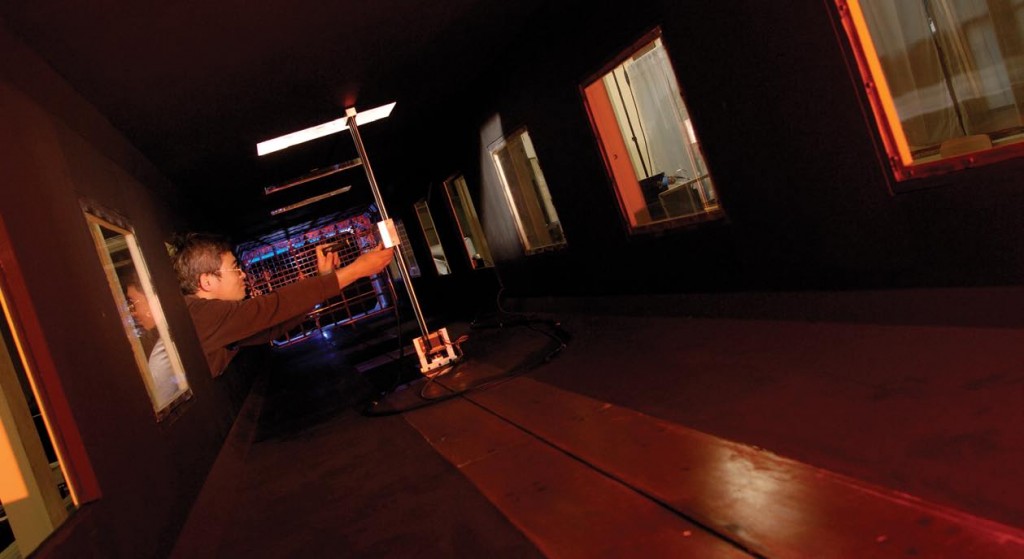
Navigating the Depths

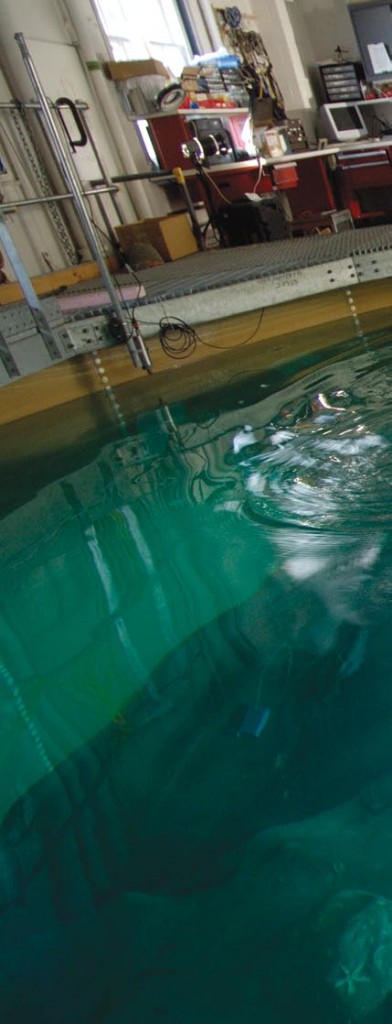
Before the two-story, 43,000-gallon Hydrodynamics Tank was constructed in Maryland Hall in 2002, researchers in Louis Whitcomb’s lab had to limit their experimental research to three-week stints at a Naval Academy lab (and occasional trips to Whitcomb’s backyard pool). “Now,” says postdoc James Kinsey, with satisfaction, “we can do work all the time.”
The theoretical work conducted by Whitcomb and company involves developing control algorithms to allow the highly precise maneuvering of deep sea robotic vehicles. To test and fine-tune their software, the mechanical engineers launch the nearly 400-pound JHU-ROV (Remotely Operated Vehicle) into the tank and send it through its paces—controlled via a bank of computers (described as the “brains of the operation”) that sit on the observation deck above the tank.
As Kinsey describes it, the work involves solving complex questions of navigation (Where are we?) and control (How do we get where we want to go—and hover there?). These are hardly idle questions when you’re remotely maneuvering a tethered underwater robot across the vast depths of the ocean floor. The goal “is to minimize surprises later when it’s actually out in the Atlantic,” Kinsey says. “With this system, we can get sub-centimeter precision,” says PhD candidate Steve Martin. Enabling a deep sea robot to hover over the sea floor reduces the amount of sediment kicked up (sediment that interferes with visibility), and avoids having the robot land on and destroy artifacts or ocean life.

This capability is key to Whitcomb’s latest project, known as Nereus, which he’s tackling in collaboration with Woods Hole Oceanographic Institute. The minivan-sized underwater vehicle is slated to descend to the ocean’s deepest depths—the Marianis Trench, 11,000 meters below sea level—in spring or summer 2008. The goal, says Whitcomb: “to provide U.S. scientists with routine access to ocean trenches, access that they don’t have right now.” (Currently the deepest diving underwater vehicle and submarine can only drop to 6,500 meters.) The “hybrid” Nereus will first fly 100 meters off the sea floor, taking sonar measurements of topography that can be assembled into a map, which scientists can use to “choose sites of interest to investigate,” explains Whitcomb. Later Nereus will be sent down again, this time equipped with a robotic arm to gather samples from the targeted sites on the trench floor.