Jennifer Elisseeff pioneers a path that could lead to repairing bone, joints, and organs in a minimally invasive way.
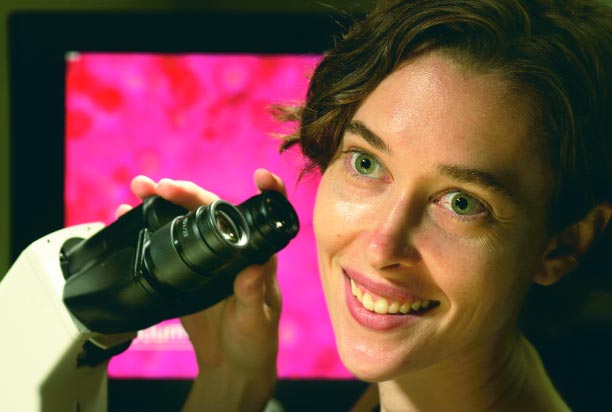
At least once a week, another letter lands on the desk of Jennifer Elisseeff. The contents are always the same—urgent requests from people who want to volunteer as test subjects for her research. “I just received one today from an older woman in Cape Cod,” says Elisseeff, shaking her head. “There’s a real need out there.”
Though an engineer by training, Elisseeff, assistant professor of Biomedical Engineering in the Whiting School of Engineering, is poised at a research door. When opened, it could mean the end of painful degenerative joint disease and related ills for countless thousands of people—and possibly, the hope of tissue-regenerated organs for millions more.
Elisseeff’s research caught the public eye when in February 2003 it was named by Technology Review as one of the “10 Emerging Technologies That Will Change the World.” The magazine published by the Massachusetts Institute of Technology noted that “Elisseeff is part of a growing movement that is pushing the bounds of tissue engineering, a field researchers have long hoped would produce lab-grown alternatives to transplanted organs and tissues.” The previous year, the same authoritative publication had named Elisseeff “one of the World’s Top 100 Young Innovators in Technology and Business.”
When Good Cartilage Goes Bad
To grasp the significance of Elisseeff’s breakthrough, we first need to understand its context—and the tough elastic stuff found in human joints, noses, and ears. In clinical terms, this human tissue known as cartilage is a connective tissue composed of cells called chondrocytes. These cells in turn are embedded in an extracellular matrix that is high in water and collagen content. Joint cartilage, which acts as a kind of shock absorber between connecting bones, can begin to wear away as an individual ages, though the cause remains a matter of debate. In some individuals, this joint cartilage can completely degrade over time, leading to osteoarthritis (OA), one of humankind’s oldest and most common diseases.
Usually diagnosed in knees or hips, OA (also known as degenerative joint disease) results in severe joint pain, loss of mobility, and even long-term disability. The only treatment, in many cases, is a major surgery involving the implantation of an artificial joint that will itself degrade over a period of years. While such orthopedic procedures have made great advances in the last decade, thanks to new implant materials and microsurgeries, the fact remains that once cartilage starts to deteriorate, that’s it—whether the culprit is OA, genetic causes, or injury. Unlike most other tissues in the human body, cartilage stubbornly refuses to regenerate naturally. As a result, in the United States alone, about 160,000 hip replacements and 266,000 knee replacements are performed yearly. These numbers are expected to rise dramatically in the next 10 years as America’s senior citizens double from 15 percent to 30 percent of the population.
In light of this age-old ailment, the discovery made by Elisseeff’s Biomaterials and Tissue Engineering Laboratory is nothing short of revolutionary. Applying research techniques developed over a decade of work, Elisseeff and her 15-member team have created a light-sensitive polymer solution that carries a payload of engineered cells and nutrients. Injected under the skin at the point of a failing joint, this mixture is then exposed to ultraviolet light, causing the polymers to harden into a stable gel-like scaffolding (called a hydrogel) that contains the cells. Over time, these encapsulated “seed cells” begin to multiply, developing into—you guessed it—cartilage. The biodegradable scaffold eventually dissolves, leaving only healthy tissue.
“Photopolymerizing hydrogels are very useful because they only harden when light is applied,” Elisseeff says. “Also, primary chondrocytes—cartilage cells—can be encapsulated in these hydrogels and will form cartilage-like tissue. The cells thrive because hydrogels contain plenty of water, which is needed to carry nutrients to these cells and move waste products away from them. The hydrogels also have enough space to allow the new tissue to form.”
“If this technique ultimately works the way we believe it will, doctors will have a new and possibly more effective option for treating severe joint injuries.”Jennifer Elisseeff
More importantly, this application works in vivo. In clinical tests with rats and mice, the injectable system generated healthy chondrocytes without a hitch. Human trials are still some years away, so she has to say “no” to those many letter-writers—at least for now. In a recent interview with Science Daily, Elisseeff expressed the hope that her system eventually will give rise to more effective treatments of joint disease or injuries. “If this technique ultimately works the way we believe it will, doctors will have a new and possibly more effective option for treating severe joint injuries,” she said. “This procedure would also help people avoid invasive surgery.”
Growing Cartilage and Bone, and a Hybrid Cornea
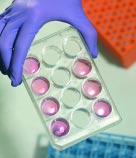
One of the most interesting features of Elisseeff’s system concerns the “payload” cells themselves. Her most recent research has involved the applied use of stem cells, a type of cell that possesses two key traits. Under proper conditions, stem cells can replicate themselves over a very long time. And when given the appropriate signals (think of booting up a new program in your computer), stem cells can differentiate and form completely different kinds of cells—and eventually tissue.
In recent months, Elisseeff’s lab has been using stem cells from the bone marrow of adult goats, along with specific growth stimulators that help direct cellular differentiation. Her group has coaxed these goat stem cells into forming either chondrocytes or osteoblasts (precursor cells that develop into bone). “This means that in the lab, we’ve already used adult stem cells to create tissue resembling cartilage by composition and morphology in the photopolymerizing hydrogel, and early tests indicate this technique may work with bones, too,” Elisseeff says.
Other tests are using adult human multipotent cells, which can be stimulated to produce different types of musculoskeletal tissue. In theory, patients preparing to undergo cartilage or bone repairs would be able to donate their own stem cells prior to the procedure, thus reducing the likelihood of infection and tissue rejection. “The approach is to see if we can try to control their differentiation,” notes Elisseeff. “Right now we’re using tissue engineering as a sort of intermediary to put the cells in biological niches, where they’re normally found in vivo, but in a controlled in vitro environment. So little is known about these cells that we could easily end up with something else.”
Although much of her research centers on orthopedics and plastic surgery applications, Elisseeff is considering other possibilities. In the past few months, she and her team have begun work with Oliver Schein at the School of Medicine’s Wilmer Eye Clinic on a synthetic hybrid cornea. “Real corneal transplants are very popular today, with more than 40,000 transplants now each year,” say Elisseeff. “However a certain number of them simply don’t work. So we’re looking at making some type of hybrid syntheticbiological implant.”
Another project involves the cartilage located between the vertebrae in the human spine. The intervertebral disk is just that— a disk-shaped cartilage pad with a gel-like interior. When these disks deteriorate, severe lower-back pain can result. Working with members of the School of Medicine’s Department of Neurosurgery, Elisseeff and her team are applying their cartilage regeneration techniques to this problem.
A Love of Lab Work Started Early
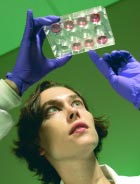
Even with the high-tech nature of her research, Elisseeff comes by her successes the old-fashioned way—through natural talent, personal drive, and a professional momentum achieved through 12 years of steady, concentrated work.
As a child in Florida, she played with microscope kits and a telescope, and later, as a teenager, worked in the lab of her father, a professor of oceanic engineering and materials science. In high school, Elisseeff took courses at a local university, went to the International Science Fair twice, and attended the Youth Science Fortnights in London, courtesy of the U.S. Navy. “I learned early on that I enjoyed working in the lab and found that it could lead to opening horizons,” she recalls.
After graduating with honors in chemistry from Carnegie Mellon University in 1993, Elisseeff entered the PhD program in biomedical engineering at the Harvard-MIT Division of Health Sciences and Technology. While working as a research assistant in the lab of her advisor, MIT’S Robert Langer, she became interested in tissue engineering, which, combined with her undergraduate experience with polymer chemistry, led to a new course of study she has pursued ever since. “During my medical studies, I started veering to a more applied pathway, trying to use these biomaterials for more specific applications,” she explains. Elisseeff’s specific concentration on cartilage began soon after, while she was working with plastic surgeons from Massachusetts General Hospital. “I remember thinking to myself, ‘Here’s a problem we need to solve,’ ” she recalls.
In 1999, with her new doctorate in hand, Elisseeff was accepted as a Pharmacology Research Associate Fellow at NIH’s National Institute of General Medical Sciences. During her year there, she continued her studies in cartilage developmental biology and tissue-engineered cartilage. “I went into this post-doc program in developmental biology thinking that I wanted to put cells and a scaffold together to see what came out of it. It was a little bit of a black box.”
She also enjoyed her interaction with fellow biologists. “Actually, it was kind of funny, because when you talk from an engineer’s perspective to basic biologists, they sometimes think you’re really crazy!” she says with a smile. “That was the neat part about it, learning a biologist’s versus a chemist’s approach to the same problem. Being realistic in the short term, what we’d like to do is to develop a treatment to delay a cartilage replacement—to improve the regeneration partially, and make someone feel better.”
Among Colleagues at Clark Hall
Since her arrival as a faculty member at Hopkins in 2001, Elisseeff has intensified her research. A visit to her lab reveals the full scope of her current studies. Housed on the first floor of the Whiting School’s new Clark Hall on the Homewood campus, Elisseeff’s facility is affiliated with the Whitaker Biomedical Engineering Institute, located in the same building. The Institute draws talent from both the Hopkins School of Medicine and the Whiting School of Engineering (especially those in the Department of Biomedical Engineering). It promotes a highly interactive environment in which multidisciplinary teams of engineers, scientists, computer scientists, and mathematicians can address biomedical challenges of the 21st century.
Supported by grants from the Arthritis Foundation as well as from several departments within the School of Medicine, Elisseeff has assembled a 15-person interdisciplinary team that includes a cell biologist, a plastic surgeon, an orthopedic surgeon, a polymer chemist, and graduate students. In each area of overlapping research—scaffold development, cell engineering, developmental biology, and clinical application—she has organized a three-tiered system of a PhD fellow or collaborator, a graduate student, and an undergraduate. “It really provides great perspective for each person involved,” she says. “The general philosophy of the laboratory is to work closely with clinicians. That gives us a better view of what is missing in some current clinical technologies. So there is a really nice bridge—good rigorous chemistry with a desired clinical application.”
Her department chair agrees. “Having a multifaceted team in biomedical engineering is the name of the game—because the discipline is a very interdisciplinary field,” says Murray Sachs, the Massey Professor and director of Biomedical Engineering. “It’s especially necessary for Jennifer’s area of research. In her case, we are at the forefront of that research.”
Insights on the Road
In her current role as the lab’s principal investigator, Elisseeff admits that she misses spending actual time working in the lab. “I try to get in and talk with people and see what’s going on,” she says, “But if I want to get anything done, I have to come in when no one else is around.”
Despite the constant avalanche of meetings, reviews, journal clubs, and deadlines, Elisseeff manages to maintain her investigative focus, even when traveling to give lectures or to tour the in-house research facilities of companies. “I find that I come up with a lot of good insights when I travel, and I have a moment to think. So, I’m always calling my lab and telling them, “Oh, I’ve got this great idea.” That’s also her quiet time to catch up on some writing—like the proposal she’s currently drafting to secure funding for the next round of her arduous research process, this time using larger animal test subjects. If all goes well, human trials will follow in a few years.
“We’re going to face new problems as we work with larger animals,” she says, a worried note in her voice. Then she brightens and laughs. “But we’re engineers—we’re supposed to solve problems.”
Visit the lab’s web site at www.bme.jhu.edu/labs/jhe/
Special thanks to Phil Sneiderman for his contributions to this article.