For Joe Katz, heading up the ambitious 10-year effort to build the Hopkins Heart, a permanent replacement heart, is just the latest challenge in a storied career.
It was at a Whiting School of Engineering retreat in the fall of 2012 when Joe Katz, the William F. Ward Sr. Distinguished Professor in Mechanical Engineering, first heard about the extraordinary plan. Duke Cameron, chief of cardiac surgery, and Gordon Tomaselli, chief of cardiology over at Johns Hopkins Medicine, had been floating an ambitious idea among colleagues of pooling the intellectual resources from Johns Hopkins’ medical and engineering communities, as well as the Applied Physics Laboratory, to successfully build something that had never before been accomplished: a permanent, internally self-sustaining, total artificial heart.
But back then, Katz knew nothing about the cardiovascular system. He’s a mechanical engineer, a specialist in fluid mechanics, who had made a name for himself by employing high-tech instruments to take measurements in a huge variety of fluid systems, from the ocean to the laboratory, with unprecedented accuracy. He’s used to testing turbines, propellers, jet engines—not blood flow. “I’m a pump guy,” says Katz matter-of-factly. But this was the pump problem to end all pump problems. “There’s no way you’re keeping me out of this,” he told Nick Jones, former dean of Engineering.
Two years later, Katz has become the Whiting School’s point person for the multi-disciplinary effort, now known as the Hopkins Heart Program, a 10-year plan to build a permanent replacement heart. The program includes cardiologists; surgeons; and mechanical, biomedical, and systems engineers from across the Johns Hopkins community, all collaborating on the project.
Artificial hearts have been around for 30 years, but building one that keeps a patient alive for longer than a few months, does not require constant monitoring or rely on burdensome external power sources, and is biologically compatible with the body, has never before been accomplished.
“It’s a very difficult problem,” says Ed Schlesinger, Benjamin T. Rome Dean of the Whiting School. “It’s a materials problem, fluid, mechanical, energy, medical. It’s got so many different components. The question is, which organization can bring together the array of expertise to address such a problem? I think, therein, Hopkins has a unique position.”
For Katz, an engineer accustomed to figuring out creative solutions to difficult problems, the Hopkins Heart Program is the biggest challenge of a storied career.
Joe Katz isn’t just a pump guy. He’s a complex turbulence guy. A multi-phase flow guy. A free-surface flow guy. But he started as a bubbles guy.
A native of Tel Aviv, Katz, 61, arrived in the United States in 1977, fresh out of Tel Aviv University and a stint in the Israeli army. When he stepped off the plane in California, it was his first time outside his homeland. “It was like being on a massive roller coaster and by the time you wake up, you find yourself on the other side of the world and you don’t remember how it happened,” says Katz, in a still thick Israeli accent. He speaks thoughtfully, his voice rising with enthusiasm whenever he makes a point.
His graduate and postdoctoral studies at Caltech focused on cavitation, the formation of bubbles in water due to changes in pressure. Using camera-mounted submersibles lowered from boats, he was among the first to use holographic imaging to investigate these micro-bubbles and their flow at the bottom of the sea.
And while he observed plenty of bubbles, he also saw something else. “When you look at the bottom of the ocean, even just a few centimeters, it is such a cesspool of life. It is absolutely amazing to see that,” he says. “You have swimming organisms, and they swim in three dimensions. If you use a regular camera and focus on a plane, they swim in and out of your plane and you don’t see them anymore. If you want to study their behavior, you need a three-dimensional imaging technique.”
When he showed the 3-D images to marine biologists, they were amazed. “When they saw my holograms, they went crazy,” he says. “They had never seen three-dimensional photographs of plankton.”
This started a new—and unlikely—collaboration with biologists using holography as a means of visualizing behavior and distribution of plankton and other micro-organisms in the oceans. Katz has since traveled all over the world on ships, using submersibles to observe how organisms interact with one another and the flow of ocean waters around them. (The irony is that Katz gets violently seasick. “Just looking at a boat makes me sick,” he says.)
In the late 1980s, Katz began to incorporate particle image velocimetry (PIV), a method of flow visualization that uses lasers to illuminate a plane within a flow field seeded with particles.
He equipped new submersibles with PIV lasers and was able to study the velocity of flow within a plane at the bottom of the ocean—something that had never before been done with such precision. At the same time, he was using PIV equipment to look at turbulent flows in his lab.
“Most people were measuring fluid velocities at a single point, but he’s perfected the ability to use PIV to take entire movies in three dimensions,” says Charles Meneveau, the Louis M. Sardella Professor of Mechanical Engineering and a longtime collaborator. “The ability to capture entire vector fields in three-dimensional space has enhanced our ability to understand turbulent flows tremendously.”
“I am always trying to advance measurement techniques,” says Katz. “Pushing the limit as much as you can, being a little bit of a daredevil. I try to build things having
no idea if they will work or not. Then we put them on the deck of a boat and find out.”
More often than not, they work out just fine.
Katz has applied his unmatched expertise in holography and PIV to secure numerous grants from the U.S. Navy and Air Force, Department of Energy, NASA and NOAA,
and private industry.
In his Laboratory for Experimental Fluid Dynamics, a series of rooms in the basement of Latrobe Hall, Katz and his students measure flow characteristics of equipment ranging from boat and submarine propellers, to a new jet pump for a naval ship, to an automotive fan for the Bosch corporation. With monies from the National Science Foundation, he and Meneveau recently measured turbulent flows over miniature model tree canopies, data that could be used for understanding atmospheric wind and ocean currents and their effects on climate change.
The goal with most projects is to collect benchmark data—information that can be used by government and industry in their own larger-scale simulations. When it comes to pumps and turbo machines, Katz’s data helps show what causes adverse effects—stalling and surging, vibrations, and noise—on the equipment.
“I’m a basic researcher,” says Katz, with typical modesty. “I’m always looking for fundamental research problems. I’m trying to understand what goes wrong.”
It’s the first question he asked cardiologists when he signed on with the Hopkins Heart Program: “What’s gone wrong with previous artificial hearts?”
The challenges of creating an artificial heart are myriad. There are problems to solve regarding biocompatibility, power supply, blood flow, pumping systems, control mechanisms. Should it be fabricated from artificial materials, muscle tissue grown from stem cells, or a combination of both? Does it have to pump like a real heart or should it rely on a system of continuous flow, like current heart-assist devices?
It’s been 32 years since William DeVries implanted the Jarvik-7 artificial heart in a patient, who lived for 112 days. In the time since, artificial hearts have become more advanced and have kept patients alive for longer periods of time, but still there isn’t one that provides a permanent solution.
Currently, there is only one total artificial heart approved by the federal government for use in patients in the United States, but it has proved only to be effective for up to 18 months and, because of its size, can only be implanted in people with large frames. In September, a French company, CARMAT, implanted its second artificial heart, made from polyurethane and natural materials derived from bovine heart tissues, in a patient. (Its first recipient died 75 days after surgery.) Tens of thousands of people are diagnosed annually with conditions that would benefit from new hearts, but because of a shortage of viable organs, only 2,000 to 2,500 transplants take place each year. Mechanical heart-assist devices help keep patients alive, serving as a “bridge” until a transplant organ can be found, but many patients die waiting.
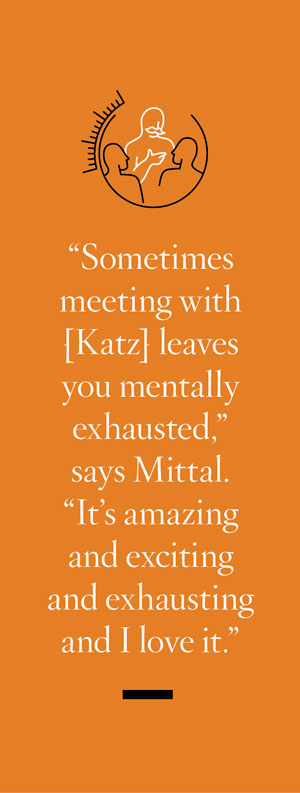
Last winter, more than 160 people from the Johns Hopkins community and beyond attended the first Hopkins Heart Symposium. The purpose was to begin the collaboration between doctors, engineers, and researchers from Johns Hopkins’ Applied Physics Lab in Laurel, Maryland. William DeVries himself served as the keynote speaker, while Johns Hopkins doctors presented talks on subjects like “Heart Failure 101” and “Stem Cells and Tissue Engineering.”
Joe Katz gave a talk simply called “How to Pump Blood.”
“There were physicians and surgeons who had never seen a pump before in their lives,” says Katz, who has participated in several subsequent brainstorming sessions with physicians. “So I wanted them to understand and to ask them: What are the options? What are the shortcomings of different designs? I want the surgeons to come back to me, and say, ‘This makes sense. This doesn’t.’ If they tell me it’s insane, wonderful! Perfectly fine. We will try again.”
Katz admits that when this project started, he didn’t know much about the heart. So he set out on a journey to gather as much information as he could, picking the brains of colleagues in engineering, meeting with cardiologists at the hospital. He sat in on open-heart surgeries and spoke with patients who use heart-assist pumps.
“Seven or eight months ago, he knew very little about cardiovascular flow,” says Rajat Mittal, a Whiting School professor in mechanical engineering and specialist in hemodynamics. “But I’d be willing to bet that he’s educated himself to the degree
that there are very few people who know more about it than he does.”
Mittal says Katz is ideally suited to head up the heart program. “He brings a level of enthusiasm to everything he does, and that’s especially true with the Hopkins Heart initiative,” he says. “Sometimes meeting with him leaves you mentally exhausted. Within a half hour, he comes up with so many new ideas, and he’s peppering you with questions, he’s disagreeing with you and agreeing with you and challenging you. It’s amazing and exciting and exhausting and I love it. I’ll take my lunch and meet
with him in his office and we’ll talk about fluid mechanics or the Hopkins Heart and
I always come back and feel like I’ve just had one of those five-hour energy drinks.”
“There’s been a lot of back-and-forth,” says Gordon Tomaselli, at Johns Hopkins Medicine. “We have no expertise in the kinds of things that Joe does in engineering and fluid dynamics and fluid flow, and he’s been a remarkable partner in all of this. He’s thought about fluid dynamics on a scale that’s quite immense—submarines and those kinds of things—and now he’s kind of shrunk his thinking to try and apply some of the same concepts about turbulence of blood flow. … I think it’s an amazing complement to the incredible body of work he’s already done.”
Katz’s first mission is to collect data on current heart-assist devices, to better understand how blood interacts with the mechanisms at the cellular level. Flow and turbulence-induced shearing cause blood clots and rupturing of red blood cells—major problems in patients with artificial hearts. Using holography and PIV techniques, Katz’s team will measure flow and shear distribution at the microscopic level, recording the damage that occurs in a pump. Then medical researchers in hemodynamics and coagulation will run plasma through the system, tagging platelets with fluorescent material. The goal is to physically witness and record where the damage occurs—basic research that needs to be accomplished before the heart project moves forward.
“We want to make sure we understand the mechanisms at that level so we don’t go design something that, right out of the box, starts to damage the blood,” says Marty Devaney, a senior administrative manager at the Whiting School who’s coordinating research efforts between Engineering, Medicine, and APL. “It’s fundamental to
the whole point. If we create an item that damages the blood, we’re no better than any system out there right now, and we want to make sure we take into account the actual mechanism that does damage to the blood and limit that in our designs. … It’s a complete, perfect collaboration between engineering and medicine, a great example of where we need to have these cross-disciplinary folks working.”
If it seems as if Joe Katz’s research interests cast an exceptionally wide net, it’s because they do. He jokes that the Hopkins Heart Program is now his night job. Just add it to the list. “Most people move from one project to another,” says Andrea Prosperetti, the Charles A. Miller Jr. Distinguished Professor of Mechanical Engineering, who was a postdoc at Caltech when Katz was a doctoral student, “but Joe keeps all of them going! He doesn’t give up the old ones. I don’t know how he does it.”
Prosperetti, an authority in the area of fluid dynamics and underwater acoustics and a member of the National Academy of Engineering, says he’s the one who recruited Katz to Johns Hopkins, when he was an assistant professor at Purdue University. “When I arrived [at Hopkins] in 1985 we were in the process of growing the department and we were thinking of who we could get. I, of course, thought of Joe, and it’s one of the handful of things of which I am the proudest, to have enticed someone like Joe to join our department. … Joe’s a giant.”
New research opportunities clearly excite Katz (“I’m like a child in a toy store,” he says. “Every day you want something else.”), but it’s working with students that gives him the most satisfaction. “I love teaching—graduate students, undergraduate students, even high school students,” says Katz. “The primary benefit of being at a university is the interaction with young students. They are smart, creative, and they are not set in their ways. It’s not surprising that most of the greatest scientific inventions have been created by young people. After you get older and more experienced, you start putting everything in your brain into little boxes, and those boxes are terrible because you need to break boundaries.”
Breaking boundaries is exactly what researchers will have to do if the Hopkins Heart Program is to be successful.
Katz knows the challenges that lie ahead, but he’s confident they will be overcome. “Look, we have already built extremely complicated systems,” says Katz, who’s been playing a key role in trying to source grants from government agencies like the National Institutes of Health as well as private donations required to fund the project. “We just need to think of it in that way—looking at the heart and cardiovascular system as a system. The challenge is in terms of materials, energy, biocompatibility, etc.”
Katz says he’s looking forward to working with colleagues across the Johns Hopkins campuses, of late nights spent brainstorming and problem-solving and proving ideas wrong and right. “I’m still grasping at the enormity of the problem,” he admits.
“I have some ideas, and I don’t know if they’re right, but if you combine Engi-
neering, Medicine, and APL all together, we have this enormous intellectual capacity, and we should take advantage of that. I believe we are going to make a dent in
this problem.”
After all, the heart is just a pump, and Joe Katz is a pump guy.
Inside the Dream Oil Spill Laboratory
Since 2011, Katz has been the co-principal investigator in the Gulf of Mexico Research Initiative (GoMRI) DROPPS (Dispersion Research on Oil: Physics and Plankton Studies) consortium, a collaboration of research universities investigating and modeling processes involved with the dispersion of oil spills and interactions of oil with marine life.
In 2012, using monies from the Deepwater Horizon settlement with British Petroleum, Katz, who had studied the interaction of diesel fuel and seawater for the U.S. Navy in the 1990s, built his “dream oil spill laboratory” in the basement of Latrobe. It’s a state-of-the-art facility with a 6-meter-long wave tank and a floor-to-ceiling glass-and-metal cylinder that researchers are using to study the interaction of seawater with subsurface oil plumes, like the one produced by Deepwater Horizon.
In the lab on a recent afternoon, postdoc David Murphy, MS ’00, was busy filling a 500-gallon tank with simulated seawater. His work involves looking at the impact of rain on oil slicks on the ocean surface and how tiny droplets can become airborne and carried by the wind—a potential environmental hazard to cleanup workers and nearby populations. Murphy says that the previous evening, he and Katz and other postdocs were working side by side in the lab well into the night—business as usual in the Katz lab.
In a sentiment that’s echoed by both his students and colleagues, Murphy says that Katz is as dedicated to his students as he is to his research. “One time he told me that the product of his work is his people. Not the work but the people,” says Murphy. “That he works on molding his students and providing a good model for them. I think that’s true. I’ve noticed that even in the midst of everything he has to do, when he’s with you, he’s really with you.”