Building a wide wave tank will give coastal engineer Robert A. “Tony” Dalrymple a dynamic tool for predicting the behavior of shorelines.
From now on, you won’t have to travel “down the ocean” from the Homewood campus to hear the sound of pounding surf. In fact, the waves will be just a few blocks away in the former Stieff Silver building, now home to the Whiting School Department of Civil Engineering’s Coastal Engineering Laboratory. The 20,000-gallon wave tank there will be the first facility of its kind at Johns Hopkins—and the only one of its design in the nation, according to the lab’s director, Robert A. “Tony” Dalrymple, the Willard and Lillian Hackerman Professor of Civil Engineering and chair of the department.
Now in its final stages of construction, the wave tank is an aquarium-like steelframed rectangular structure 58 feet long, 8 feet wide, and 6 feet tall. At one end of the tank will sit the “wave maker,” a motorized set of four paddles that can be programmed independently to operate at varying speeds. The tank’s floor gently rises to the opposite end, simulating a sloping beach and constructed of plastic panels on an aluminum frame. Overhead, a steel T-rail runs the full length along one side of the tank, and a 30-foot linearrail set runs along the other. Two remotely controlled instrument carts will track wave motion below.
To understand this wave tank’s unique properties, says Dalrymple, you first need to know the difference between a wave basin and a wave tank, both of which are used by coastal engineers to investigate the hydrodynamics of wave action. “Wave basins are very large, three-dimensional structures that allow you to send waves in at different directions,” he notes, “while a wave tank tends to be two-dimensional because it’s very narrow. However, our wave tank is one of the widest in the U.S., and uses four paddles instead of the usual one to generate wave action.” As a result, the hydraulic piston-actuated paddles can be phased to generate either a single wave traveling straight down the tank or a series of waves moving at different angles and frequencies, says Dalrymple. “Each wave can have a different direction associated with it, so we can generate a fairly realistic sea state,” he explains. “In other words, our tank is very versatile. It can simulate the properties of a 3-D wave basin in a two-dimensional tank.” To be precise, it’s 2.5-D, according to graduate student Asher Peltz who is in charge of the construction and the data analysis to come. Funding for the wave tank research comes from Johns Hopkins and from the Office of Naval Research.
When Shorelines Suddenly Shift
Why study waves? In the complex and often theoretical discipline of coastal engineering, there are some very solid reasons. The field itself focuses on two interrelated studies—wave mechanics and sediment transport. Both are key in understanding and, to a certain extent, predicting the course of shifting shorelines due to sudden storms or slow attrition.
“Controlling beach erosion—that’s the big Holy Grail of what we do as coastal engineers,” says Dalrymple. “Our goal is to be able to predict the behavior of a shoreline over both short and long periods of time. If a northeaster comes to Ocean City, Maryland, tomorrow, we want to be able to predict what the beach will look like after that storm—and whether to evacuate people, even when we have only a day or two of advance notice.”
By contrast, long-term forecasting focuses on the possible impact of major shoreline projects (such as the modification of an inlet or the construction of a large, beach-front building) over a period of years. Dalrymple notes that this type of forecasting also has a direct application for the petroleum industry, in assessing the construction of offshore drilling platforms and large tanker vessels and how both interact with ocean waves.
Into the “Jaws” Surfers Love
In a sense, Tony Dalrymple has always been looking beneath the waves for answers. As an engineering undergraduate at Dartmouth in the late 1960s, he imagined himself building deep-sea human habitats. Then, as a graduate student studying in conducive locales like Hawaii and Florida, Dalrymple found his true calling. After a 29-year teaching stint at the University of Delaware, during which time he founded and directed its renowned Center for Applied Coastal Research, he joined the Whiting School in 2002 as the Hackerman Professor and chair (in 1999-2000 he was a visiting professor at the Whiting School). Considered to be one of the foremost researchers in the field of coastal engineering and wave mechanics, he is often sought out for his expertise on wave-related issues, including one that he laughingly refers to as “my 15 minutes of fame!”
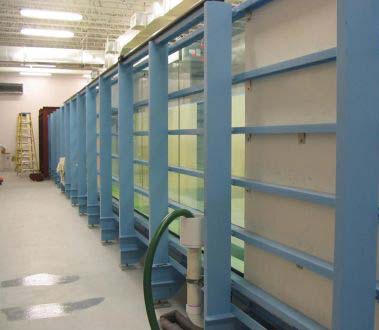
In 1998, during the preparation of a cover story, National Geographic researchers approached Dalrymple with a request— would he be willing to take a look at “Jaws?” In this case, “Jaws” was not a ravenous shark, but a famed surfing spot just off the northern beaches of Hawaii’s Maui Island. What the magazine wanted Dalrymple to explain was why suddenly, and for no apparent reason, the waves at Jaws would swell as high as 70 feet. While this phenomenon delighted surfers, its cause remained a mystery.
Consulting a hydrographic survey, showing depth measurements in that area, Dalrymple input these data into a wave-modeling program that he and another colleague had developed at the University of Delaware. Right away, he saw that the basis for the huge waves was very simple. A submerged ridge formed by ancient lava flows caused the ocean floor at Jaws to rise rapidly from 120 feet to 30 feet. While normal waves passing over the ridge were unaffected, more powerful storm swells moving from deep sea to shore “really tripped over the ridge,” says Dalrymple. “These big storm waves shoaled up and refracted on this ridge, which focused them to a point.” The result of this refraction—pinching the wave so that its power is directed inward and upward—was one monster wave after another. Dalrymple’s solution to the Jaws mystery was featured in the October 1998 issue of National Geographic and discussed in a report on CNN.
Breaking Down Waves by the Numbers
These days, Dalrymple is more than content to pursue his core interest in studying wave dynamics. Aside from analyzing wave action in his lab’s new wave tank, he is also looking at other research possibilities through computer-based modeling. “The theoretical basis of what I do has changed enormously over the last 30 years,” he notes. “The sophisticated numerical tools and computing power that we can bring to bear on problems now are much more powerful than previously.”
As examples, Dalrymple cites two programs he has developed. One, a Java software applet he calls the “Northeaster Risk Index” JOHNS HOPKINS ENGINEER SUMMER 2004 17 lets the investigator assess the risk potential of a northeaster storm’s impact in breaching coastal dunes and flooding inland. “The program’s predicative modeling is done empirically, based on historical data,” he says. “But I’d like to do the same modeling with real-time data and be able to predict that kind of behavior on the fly.”
The second, more sophisticated, wave model takes a page from astrophysics, specifically Smoothed Particle Hydrodynamics (SPH). As Dalrymple explains it, most wave models of the past are bound by the limitations of their mathematical theories, evidenced by the crudeness of the models themselves when they are replicated in a digital environment. However, SPH, which was used originally to model the dynamics of a galaxy and the stars within it as interactive particles, shows great promise. According to Dalrymple, “SPH gives a more accurate way of depicting wave action, including splashing, by tracking each particle of water and seeing where it goes. We’re one of the few groups in the country working with this technique.”
However, there is a drawback—the need for immense computing power in generating these models. At present, Dalrymple is limited to models using up to 100,000 particles, but hopes eventually to create models composed of millions of them.
Even as his research continues to evolve, Dalrymple can still marvel at the complex challenges presented by a simple ocean wave. “When you look at a breaking wave, all you see is a lot of foam and turbulence,” he says. “But if you tried to describe that mathematically, you would throw up your hands. There’s a lot of organization inside of a breaking wave, from activity resembling internal tornadoes to vortices on its surface. Where these come from, nobody knows. What we’re interested in is the science that explains how a wave that has no turbulence in its structure suddenly develops that turbulence when it breaks. We have theories that take waves right up to breaking, but when they do break, all bets are off.”
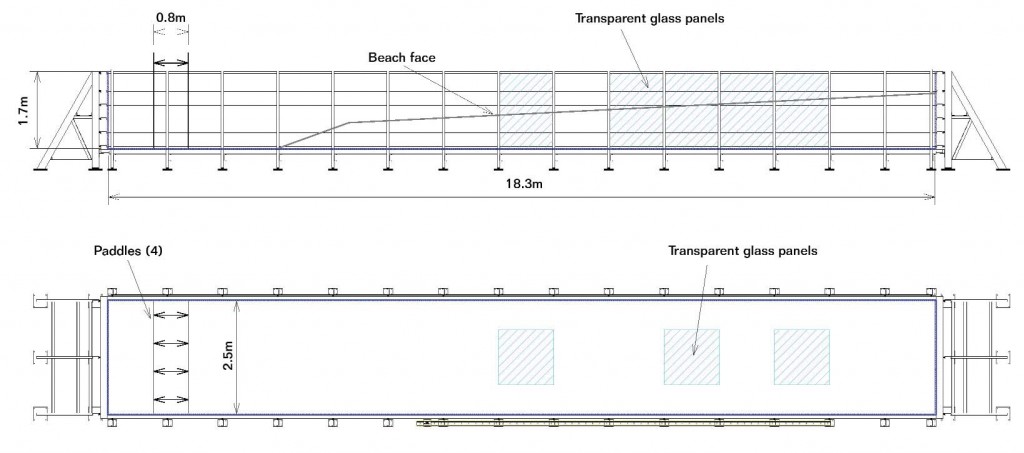
For photos of the wave tank’s construction, visit the Coastal Engineering Laboratory at www.ce.jhu.edu/apeltz/wavelab.html