Master and commander of advanced oceanic robots, Louis L. Whitcomb has set his course on the most difficult challenge yet: how to probe the least accessible places on Earth.
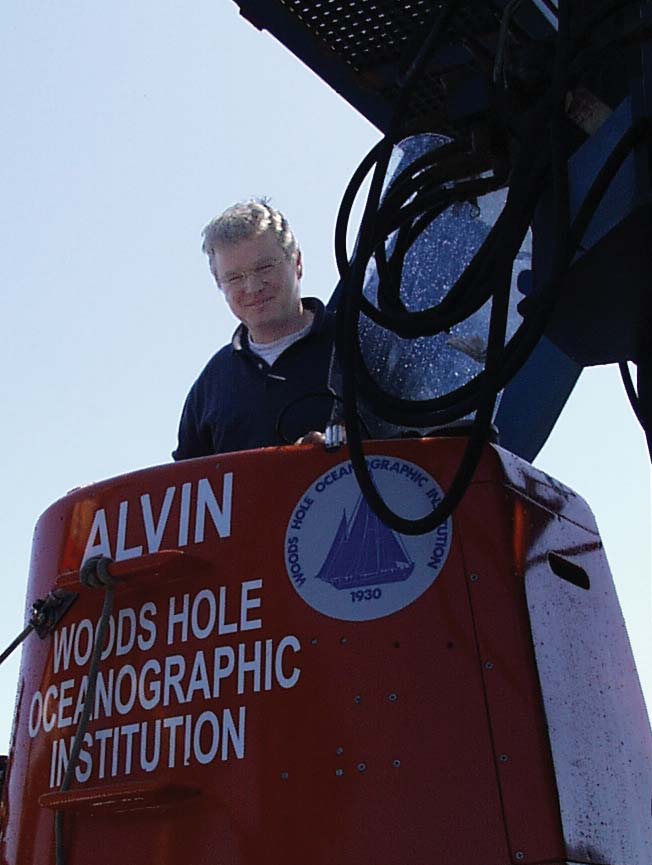
Move over, Captain Nemo. Louis L. Whitcomb and his fellow researchers at the Dynamical Systems and Control Laboratory (DSCL) are preparing to plumb new depths in deep ocean exploration that would have astounded Jules Verne. As the director of DSCL, Whitcomb, professor in the Whiting School’s Department of Mechanical Engineering, is designing new control and navigation solutions that will allow human beings to explore the deepest parts of this vast, aquatic frontier.
And what a frontier it is. It covers more than two-thirds of the Earth, with an average depth of more than 2 miles. Its deepest area is the 7-mile Marianas Trench in the South Pacific, an underwater abyss that could easily swallow Mt. Everest. Even more amazing is the fact that less than 10 percent of the world’s ocean floor has ever been explored. As U.S. Department of Commerce Deputy Secretary Samuel W. Bodman observed in 2001, “We know more about the back of the Moon than about trenches in the Atlantic.”
The Robotic Option
From Whitcomb’s perspective, the real challenge in exploring Earth’s oceans lies in developing new means for getting there. Even the most sophisticated scuba diving gear is no match for the incredible pressures exerted by the ocean’s depths, which can reach more than 8 tons per square inch. “Recreational divers might dive down to about 50 meters (164 feet), while commercial divers who use exotic gas mixes can operate down to a couple of hundred meters, but it takes a great deal of acclimation,” he notes. “The human body itself wasn’t designed to operate in the deep ocean environment.”
By the 1960s, the first deep-diving submersibles had made their appearance—and headlines—when the bathyscaphe Trieste reached a deep diving record in the Marianas Trench of 11,033 meters (36,200 feet). While such expeditions spark the imagination, they are, according to Whitcomb, ultimately impractical. “Building these kinds of ultra-deep-diving subs is very expensive and cumbersome,” he says. “Although the Trieste went to the bottom of the Marianas Trench in 1960, nobody’s been back there since. Today, only a half dozen deep-diving submersibles are in operation around the world that allow humans to explore the sea floor below 4,000 meters directly. That’s a fairly small keyhole for the hundreds of thousands of natural scientists who want access to the ocean floor.”
A viable alternative to occupied submersibles, says Whitcomb, involves the ongoing focus of his research—robotic vehicles. Known at ROVs (Remotely Operated Vehicles), they provide a much more flexible and cost-effective platform for deep ocean exploration, tethered to a surface mother ship by a cable that provides both power and communications, including live video. Unlike manned submersibles, robotic ROVs have greater mission endurance and can operate around the clock. More importantly, they offer a more open window of access for sea floor research by providing a real-time telepresence for any number of researchers topside.
The only drawback to their use in deep ocean exploration is the limit to which they can dive. JASON II, an advanced ROV launched in 2002 at the Woods Hole Oceanographic Institution in Massachusetts, is currently the deepest diving oceanographic vehicle in the world, having reached a depth of 6,500 meters (21,325 feet). “That’s a very critical level,” says Whitcomb.
“Achieving that depth gives scientists routine access to 97 percent of the ocean floor. Still, JASON II cannot reach those deepest points in the oceanic basins.”
Control Systems to Go
Whitcomb should know—as an adjunct scientist at Woods Hole’s Deep Submergence Laboratory (DSL), he and his Whiting School students were responsible for initially developing the navigation and control systems now employed by JASON II, as well as by a number of other deep-ocean ROV initiatives around the world. And it was Whitcomb and a Woods Hole colleague, Dr. Dana Yoerger, who had designed the “brains” for the original Jason, and then in 1997 outfitted the robot with a bottom-lock Doppler sonar device to enable it to operate on a pre-programmed course. On an expedition to the Mediterranean Sea that year, he was thrilled to find out how well Jason performed in photographing ancient wrecks in deep water and retrieving artifacts.
In Whitcomb’s lab at the Whiting School, researchers deploy a small, tethered, robotic vehicle, dubbed the JHUROV, in a 43,000-gallon hydrodynamics tank constructed in Maryland Hall. JHUROV was designed and built by David Smallwood ’99 MS, ’03 PhD. By putting the JHUROV through its underwater paces, Whitcomb and his team can easily test new versions of their control and navigation systems within an ideal environment.
However, creating such systems is no easy matter. To meet researchers’ needs for exact control, submersible ROVs must move precisely on command from a certain starting point at a specific heading, depth, and speed to a set destination, then recalculate those parameters instantly for the next leg of their mission. To create such controls, says Whitcomb, “We mathematically model the way a vehicle moves and try to understand the dynamics of how it responds to forces applied to it by thrusters and control surfaces. Then we build computer models of that and incorporate them into a control system that has the properties of informing the vehicle what you want it to do. The control system takes care of computing what forces and torques need to be applied to the vehicle and executes them. It’s like a cruise control, but obviously a bit more complicated.”
Navigation systems require the same accuracy. To accomplish this goal collaboratively, Whitcomb’s lab and Woods Hole have created DVLNAV, an interactive program for precision 3-D navigation of underwater vehicles. Employing such sensor technologies as Doppler sonar and a gyro compass, this navigation software, which runs on a Windows-based desktop, provides an interactive user interface with real-time displays of all sensor information and status indicators, as well as a moving map display with a real-time vehicle outline.Oceanographic researchers can access the software at robotics.me.jhu.edu/~llw/dvlnav/.
“We try to make some of the tools we’ve developed available to other universities and institutions,” notes Whitcomb. “This works out really well for us; if we want to conduct a full-scale experiment in the ocean, then we can team up with one of the groups that is already using our software.”
Reaching New Depths
With all he has accomplished, Whitcomb is finding his greatest and most compelling test may be just beginning. Through $5 million in funding provided by the National Science Foundation, the U.S. Navy, and the National Oceanic and Atmospheric Administration, Woods Hole’s Deep Submergence Laboratory and Whitcomb’s lab are set to develop an entirely new prototype of submersible ROV. The four-year project will be led by Woods Hole’s Andrew Bowen, with Woods Hole’s Yoerger and Whitcomb as co-investigators.
This hybrid remotely operated vehicle, or HROV, will be capable of performing a variety of tasks, including exploring the most remote depths of the ocean, to a depth of 11,000 meters. The advanced, battery-powered vehicle will be able to operate either autonomously to survey large areas, or tethered to get close up for samples and other tasks. Its novel fiber optic micro cable, no thicker than a fishing line, will transmit live data and video—a major improvement over the large awkward cables previously used. The HROV’s other new technologies will include ceramic housings for cameras and other electronic equipment to withstand the deep-sea pressures.
With a goal of undergoing initial trials in three years for the HROV, clearly Whitcomb and his team have their work cut out for them. Their primary focus will be to enhance existing control and navigation systems to produce computer hardware and software to be installed on the hybrid vehicle itself. “With the new vehicle, we have to solve a different set of engineering problems than we solved with JASON II,” says Whitcomb. “This vehicle has to be very light, withstand enormous pressures, and be self-powered. We have an idea about how to build this system, but we’ve never done it before.”
If successful, the HROV will provide routine access for scientific research in areas of the ocean that are virtually unexplored, including the deep trenches (some of Earth’s most active earthquake zones), as well as deep transform faults and beneath the polar ice caps. The HROV’s compact size will enable it to be deployed rapidly from almost any ship to provide data on volcanic eruptions, earthquakes, and other environmental changes.
Downward to the Next Frontier
Despite the project’s steep learning curve, Whitcomb obviously relishes his prospects for success. “If we can accomplish this project, we’d like to look at other areas that we have even less access to— for example, under the Arctic ice pack, or in the southern oceans where huge swells make research difficult,” he says.
“I’m interested in developing vehicles that can explore these marginal environments,” Whitcomb affirms with a grin. “There are enough untouched places on the ocean floor that we’re not going to run out of them any time soon, at least in my research lifetime.”
Visit the Dynamical Systems and Control Laboratory web site at robotics.me.jhu.edu/dscl
Special thanks to Phil Sneiderman for his contributions to this article.